For the first time, observations with ESO’s Very Large Telescope (VLT) have revealed that a star orbiting the supermassive black hole at the heart of the Milky Way travels exactly as Einstein’s general theory of relativity predicts. Its orbit is formed like a rosette, not an ellipse, as Newton’s theory of gravity predicted.
This long-awaited conclusion was made possible by increasingly precise measurements taken over nearly 30 years, allowing scientists to unravel the mysteries of the giant lying at the galaxy’s center.
Astronomers had only indirect evidence for supermassive black holes for many years, the most compelling of which was the discovery of quasars in distant active galaxies.
Quasars emit approximately a trillion times as much energy as our Sun from a region the size of the Solar System, according to observations of their energy output and fluctuation timelines. The conversion of gravitational energy into light by a big black hole is the only mechanism capable of producing such massive amounts of energy.
“Einstein’s General Relativity predicts that bound orbits of one object around another are not closed, as in Newtonian Gravity, but precess forwards in the plane of motion. This famous effect first seen in the orbit of the planet Mercury around the Sun was the first evidence in favor of General Relativity. One hundred years later we have now detected the same effect in the motion of a star orbiting the compact radio source Sagittarius A* at the center of the Milky Way. This observational breakthrough strengthens the evidence that Sagittarius A* must be a supermassive black hole of 4 million times the mass of the Sun,” says Reinhard Genzel, Director at the Max Planck Institute for Extraterrestrial Physics (MPE) in Garching, Germany, and the architect of the 30-year-long program that led to this result.
Sagittarius A* and the dense cluster of stars around it, which are 26,000 light-years from the Sun, present a unique laboratory for studying physics in an otherwise uncharted and intense regime of gravity.
S2, one of these stars, approaches the supermassive black hole at a distance of less than 20 billion kilometers (120 times the distance between the Sun and Earth), making it one of the nearest stars yet discovered in orbit around the huge giant. S2 is speeding through space at approximately three percent the speed of light as it approaches the black hole, completing one orbit every 16 years.
“After following the star in its orbit for over two and a half decades, our exquisite measurements robustly detect S2’s Schwarzschild precession in its path around Sagittarius A*,” says Stefan Gillessen of the MPE, who led the analysis of the measurements published today in the journal Astronomy & Astrophysics.
Observations of material around the centers of galaxies have lately provided direct evidence for the existence of supermassive black holes. If these stars and gas are being propelled by a big object with a strong gravitational field that is contained within a tiny region of space, such as a supermassive black hole, their high orbital velocities are easily explained.
Because most stars and planets have non-circular orbits, they move closer and farther away from the object they’re rotating around. S2’s orbit precesses, meaning that the location of its closest point to the supermassive black hole varies with each turn, generating a rosette structure.
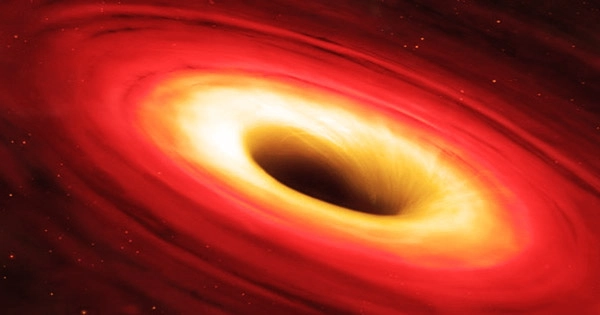
After following the star in its orbit for over two and a half decades, our exquisite measurements robustly detect S2’s Schwarzschild precession in its path around Sagittarius A*.
Stefan Gillessen
General Relativity gives a precise forecast of how much its orbit changes and the most recent observations from this study match the theory perfectly. This phenomenon, called Schwarzschild precession, had never been observed before in a star orbiting a supermassive black hole.
Scientists can learn more about the proximity of the supermassive black hole at the center of our galaxy by using ESO’s VLT.
“Because the S2 measurements follow General Relativity so well, we can set stringent limits on how much invisible material, such as distributed dark matter or possible smaller black holes, is present around Sagittarius A*. This is of great interest for understanding the formation and evolution of supermassive black holes,” said Guy Perrin and Karine Perraut, the French lead scientists of the project.
This conclusion is the outcome of 27 years of observations of the S2 star, the majority of which were conducted using a fleet of sensors at ESO’s VLT in Chile’s Atacama Desert.
The quantity of data points used to determine the star’s position and velocity demonstrates the new research’s thoroughness and accuracy: the researchers used the GRAVITY, SINFONI, and NACO instruments to make over 330 observations in all. Because S2 takes years to orbit the supermassive black hole, it was critical to track the star for nearly three decades in order to understand the complexities of its orbit.
A multinational team led by MPE’s Frank Eisenhauer collaborated on the study with researchers from France, Portugal, Germany, and ESO. The GRAVITY cooperation, called after the equipment they created for the VLT Interferometer, which combines the light from all four 8-meter VLT telescopes into a super-telescope, is made up of the researchers (with a resolution equivalent to that of a telescope 130 meters in diameter).
In 2018, the same researchers saw another effect predicted by General Relativity: light from S2 was stretched to longer wavelengths when the star approached Sagittarius A*.
“Our previous result has shown that the light emitted from the star experiences General Relativity. Now we have shown that the star itself senses the effects of General Relativity,” says Paulo Garcia, a researcher at Portugal’s Centre for Astrophysics and Gravitation and one of the lead scientists of the GRAVITY project.
The team expects that with ESO’s future Extremely Large Telescope, they will be able to spot fainter stars orbiting even closer to the supermassive black hole.
“If we are lucky, we might capture stars close enough that they actually feel the rotation, the spin, of the black hole,” says Andreas Eckart from Cologne University, another of the lead scientists of the project.
Astronomers would be able to quantify the two quantities that constitute Sagittarius A*, spin and mass, and define space and time around it. “That would be again a completely different level of testing relativity,” says Eckart.