The development of synthetic receptors for precise cell control is an exciting area of research with implications for a variety of fields, including medicine and biotechnology. Engineered synthetic receptors can recognize specific molecules or signals and initiate desired cellular responses.
A groundbreaking new technique for engineering biosensors that respond sensitively to specific biomolecules has been developed by scientists, enhancing cell migration and targeting in cancer treatment. The findings could pave the way for more precise control of cellular processes in a variety of therapeutic applications.
Biosensors are artificial molecular complexes designed to detect the presence of target chemicals or even biomolecules. As a result, biosensors have become important in diagnostics and synthetic cell biology. However, traditional methods for engineering biosensors focus on optimizing interactions between static binding surfaces, and current biosensor designs can only recognize structurally well-defined molecules, which can be too rigid for “real-life” biology.
“We developed a novel computational approach for designing protein-peptide ligand binding and applied it to engineer cell-surface chemotactic receptors that reprogrammed cell migration,” says EPFL professor Patrick Barth. “We believe that our work will have a broad impact on the design of protein binding and cell engineering applications.”
We developed a novel computational approach for designing protein-peptide ligand binding and applied it to engineer cell-surface chemotactic receptors that reprogrammed cell migration. We believe that our work will have a broad impact on the design of protein binding and cell engineering applications.
Patrick Barth
The new biosensors developed by Barth’s group can detect flexible compounds and trigger complex cellular responses, opening up new applications for biosensors. In contrast to traditional static approaches, the researchers developed a ‘computational framework,’ which is a computer-based system, for designing protein complexes that can change shape and function dynamically. The framework can look at previously unexplored protein sequences to find new ways for the protein’s groups to be activated, even if they are not normally activated.
The researchers used their new method to create synthetic receptors that can sense and respond to multiple natural or engineered molecular signals, allowing for optimal sensing of flexible ligands and strong allosteric signaling responses, which occur when a molecule binds to a different site on a protein, causing a change in the protein’s shape and activity at a different site.
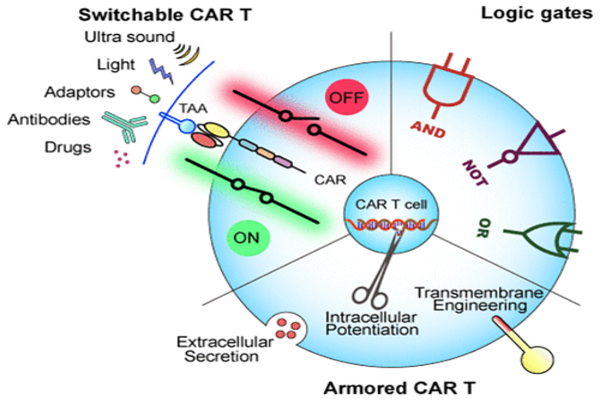
Like natural receptors, the designed receptors interact with the flexible ligands via allosteric triggers, but they improve and rearrange how the signals are transferred, similar to dialing the same number from a different cell phone with better service. The triggers appear to funnel signals through the same set of “transmission hubs” as the natural ones, but significantly improve signal transmission via optimally rewired dynamic couplings.
The findings suggest that combining a flexible sensing layer with a robust signal transmission layer is a common feature of G protein-coupled receptors, a family of enormously important receptors in the cell that are involved in almost every major aspect of its life and function.
“We were able to leverage our biosensor design to drive cell migration in lymphocytes, which migrate more efficiently towards chemokines when equipped with designed biosensors,” says Rob Jefferson, the study’s first author. “Chemokines serve as chemical beacons for immune cell recruitment in the body, a suboptimal process in certain diseases that could be improved with our biosensors.”
The new synthetic receptor design method could be useful in a variety of therapeutic contexts. Engineered cytotoxic lymphocytes with enhanced chemotaxis toward tumor sites, for example, could be useful in cancer treatment. Creating receptors that can sense and respond to specific signals is a promising new synthetic cell biology tool that could lead to more precise control over cellular processes for a variety of therapeutic applications.