A group of international scientists went back to the formation of the solar system 4.6 billion years ago to gain new insights into the cosmic origins of the periodic table’s heaviest elements. The study, led by scientists from the International Research Network for Nuclear Astrophysics (IReNA) and the Joint Institute for Nuclear Astrophysics — Center for the Evolution of the Elements (JINA-CEE), was published in the journal Science in the most recent issue.
Heavy elements like iron and silver, which we encounter in our daily lives, did not exist at the beginning of the universe, 13.7 billion years ago. They were formed over time by nuclear reactions known as nucleosynthesis, which combined atoms. Some of the heaviest elements, including iodine, gold, platinum, uranium, plutonium, and curium, were created by a type of nucleosynthesis known as the rapid neutron capture process, or r process.
For decades, the question of which astronomical events can produce the heaviest elements has remained a mystery. The r process is thought to occur during violent collisions between two neutron stars, between a neutron star and a black hole, or during rare explosions after massive stars die. Such high-energy events are extremely rare in the universe. Neutrons are incorporated into the nucleus of atoms and converted into protons as a result. Because elements in the periodic table are defined by the number of protons in their nucleus, the r process captures more neutrons, resulting in heavier nuclei.
Researchers went back to the formation of the solar system 4.6 billion years ago to gain new insights into the cosmic origin of the heaviest elements on the periodic table.
Some of the nuclei produced by the r process are radioactive and must decay over millions of years to become stable nuclei. Iodine-129 and curium-247 are two such nuclei that were created before the sun formed. They were incorporated into solids that eventually became meteorites and fell to the earth’s surface. The radioactive decay produced an excess of stable nuclei within these meteorites. This excess can now be measured in laboratories to determine the amount of iodine-129 and curium-247 present in the solar system just before its formation.
Why are these two r-process nuclei so unique? They have an unusual property in common: they decay at nearly the same rate. In other words, the ratio of iodine-129 to curium-247 has remained constant since their creation billions of years ago.
“This is an amazing coincidence, especially given that these nuclei are two of only five radioactive r-process nuclei that can be measured in meteorites,” says study leader Benoit Côté of the Konkoly Observatory. “By freezing the iodine-129 to the curium-247 ratio in time, like a prehistoric fossil, we can get a direct look into the last wave of heavy element production that built up the composition of the solar system and everything within it.”
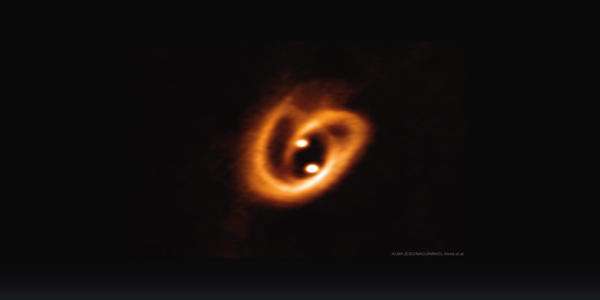
Iodine, with its 53 protons, is easier to produce than curium, which has 96 protons. This is due to the fact that it takes more neutron capture reactions to reach the higher number of protons in curium. As a result, the iodine-129 to curium-247 ratio is highly dependent on the amount of neutrons available during their formation.
The researchers calculated the iodine-129 to curium-247 ratios produced by collisions between neutron stars and black holes to determine the optimal set of conditions for reproducing meteorite composition. They came to the conclusion that the number of neutrons available during the last r-process event before the birth of the solar system could not have been excessive. Otherwise, too much curium would have been produced in comparison to iodine. This implies that extremely neutron-rich sources, such as matter ripped from the surface of a neutron star during a collision, were unlikely to play a significant role.
So, what caused these r-process nuclei to form? While the researchers were able to provide new and insightful information about how they were created, they were unable to pinpoint the nature of the astronomical object that created them. This is due to the fact that nucleosynthesis models are based on uncertain nuclear properties, and it is still unclear how to link neutron availability to specific astronomical objects like massive star explosions and colliding neutron stars.
“However, the ability of the iodine-129 to curium-247 ratio to peer more directly into the fundamental nature of heavy element nucleosynthesis is an exciting prospect for the future,” said Nicole Vassh, coauthor of the study at the University of Notre Dame.
Advances in the fidelity of astrophysical simulations and understanding of nuclear properties could reveal which astronomical objects created the heaviest elements of the solar system using this new diagnostic tool.
“Such studies are only possible when a multidisciplinary team is assembled, with each collaborator contributing to a different piece of the puzzle. The JINA-CEE 2019 Frontiers meeting provided the ideal setting for formalizing the collaboration that resulted in the current outcome “Côté stated.