The universe was a heated soup of fundamental particles called quarks and gluons for a few millionths of a second after the Big Bang. Those particles began to cool a few microseconds later, becoming protons and neutrons, the building constituents of matter.
The quark-gluon plasma (QGP) is an interacting confined assembly of quarks and gluons that is in thermal (local kinetic) and chemical (abundance) equilibrium. The word plasma denotes the presence of unrestricted color charges. Quarks are completely trapped within protons and neutrons, which is a phenomenon we don’t fully comprehend.
Around the last decade, physicists all over the world have been attempting to recreate that soup, known as quark-gluon plasma (QGP), by smashing atom nuclei together at trillion-degree temperatures. Léon van Hove noted the equivalency of the three terms: quark-gluon plasma, quark matter, and a new form of matter in a 1987 overview.
The pressure exhibits the relativistic Stefan-Boltzmann format defined by the fourth power of temperature and several practically mass-free quark and gluon constituents because the temperature is above the Hagedorn temperature and hence above the scale of light u,d-quark mass.
The early universe was extremely hot, but it was almost devoid of net-baryons. The QGP may exist even now in the interior of compact stellar objects such as neutron stars, at the opposite limit of low temperature and high baryon density.
The fundamental goal of modern nuclear physics is to construct the QGP in the laboratory under controlled settings by colliding heavy nuclei at ultra-relativistic energy. Compressing or heating nuclear materials to the point where neutrons and protons begin to overlap can form such a massive system. As the barriers between each neutron and proton vanish, a massive region of a new state of matter, quark-gluon plasma, should emerge.
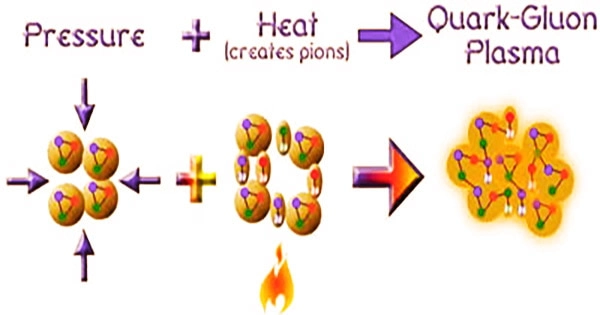
Despite the fact that quarks and gluons are made up of protons and neutrons, they behave quite differently from the heavier particles. Quantum chromodynamics, established in part by MIT academics Jerome Friedman and Frank Wilczek, who both received Nobel prizes for their work, governs their interactions.
QGP can be defined as a new phase of strongly interacting matter that expresses its physical features in the form of almost massless gluons and quarks with nearly free dynamics. For a new state of matter to be referred to as QGP, both quarks and gluons must be present in conditions near chemical (yield) equilibrium with their color charge open.
However, because quarks and gluons are contained within heavier particles, studying their true behavior is challenging. The only place in the cosmos where QGP exists is within high-speed accelerators, where it exists for barely a fraction of a second. The strong interactions between quarks and gluons dominate the features of the QGP, while the correlated vacuum’s influence is greatly diminished due to the increased volume of the system.
Nuclear collisions produce different temperatures and net-baryon densities depending on the bombarding energy. They interpolate between the early universe’s severe circumstances on the one hand and compact star objects on the other.
Under extremely high energy densities, the elementary particles that make up the hadrons of baryonic matter are freed of their strong attraction for one another, resulting in quark-gluon plasma (QGP). The lower yield of particles at high transverse momenta (pt) is the strongest evidence for the QGP.
These particles are mostly produced by infrequent, high-momentum collisions between quarks and gluons (partons) in the reaction’s early stages. In relativistic heavy-ion collisions, the QGP expands, cools, and eventually transforms into a hadronic gas. The hadronic system is so diluted at this point that maintaining equilibrium during evolution would be difficult.
Scientists at Brookhaven National Laboratory’s Relativistic Heavy Ion Collider reported generating QGP in 2005 by smashing gold atoms together at almost the speed of light. These collisions can generate temperatures of up to 4 trillion degrees, which is 250,000 times hotter than the sun’s core and hot enough to melt protons and neutrons into quarks and gluons.
The study of the QGP also serves as a test bed for finite temperature field theory, an area of theoretical physics aimed at understanding particle physics under extreme temperatures. The ensuing super-hot, super-dense glob of matter, which measures roughly a trillionth of a centimeter across, may provide fresh insights into the features of the very early universe.
So far, they’ve discovered that QGP is a nearly frictionless liquid, rather than the gas that physicists had predicted. High-momentum partons fracture into hadrons outside the collision zone, and any energy loss in the plasma softens the hadronic spectrum, lowering the measured yield of hadrons at high-pt.
Scientists want to learn more about the features of quark-gluon plasma and whether it becomes gas-like at greater temperatures by performing higher-energy collisions. A compelling case for the QGP’s existence and attributes must be based on a diverse set of observations.