A quantum speed limit (QSL) is a limitation on the minimum time for a quantum system to evolve between two distinct states in quantum mechanics. QSL are inextricably linked to time-energy uncertainty connections.
Semiconductor technology is getting quicker and faster, but at some point, physics will prevent any further advancement. Even if the material is excited optimally using laser pulses, the speed cannot be raised beyond one petahertz (one million gigahertz).
What is the speed limit of electronics? When computer processors function with smaller and smaller signals and time intervals, they eventually reach physical constraints. The quantum-mechanical mechanisms that allow electric current to be generated in a semiconductor material are time-consuming. This slows down signal creation and transmission.
These constraints can now be explored by researchers at TU Wien (Vienna), TU Graz, and the Max Planck Institute of Quantum Optics in Garching: Even when the material is excited optimally using laser pulses, the speed cannot be raised beyond one petahertz (one million gigahertz). This finding has now been published in the peer-reviewed journal Nature Communications.
Materials that do not conduct electricity at first are researched. These are hit by an ultra-short laser pulse with an extreme UV wavelength. This laser pulse raises the energy level of the electrons, allowing them to move freely for the first time. As a result, the laser pulse temporarily converts the material into an electrical conductor.
Prof. Joachim Burgdörfer
Fields and currents
Electric current and light (or electromagnetic fields) are inextricably related. This is also true in the field of microelectronics: Electromagnetic fields are used to control electricity in microchips. For example, an electric field can be given to a transistor, and depending on whether the field is turned on or off, the transistor will either allow or block electrical current. An electromagnetic field is thus turned into an electrical signal in this manner.
Laser pulses, the quickest and most accurate electromagnetic fields accessible, are utilized instead of transistors to test the limitations of this conversion of electromagnetic fields to current.
“Materials that do not conduct electricity at first are researched,” explains Prof. Joachim Burgdörfer of the Institute for Theoretical Physics at TU Wien. “These are hit by an ultra-short laser pulse with an extreme UV wavelength. This laser pulse raises the energy level of the electrons, allowing them to move freely for the first time. As a result, the laser pulse temporarily converts the material into an electrical conductor.” Once there are freely moving charge carriers in the material, they can be pushed in a specific direction by a second, somewhat longer laser pulse. This generates an electric current, which may subsequently be monitored using electrodes on opposite sides of the material.
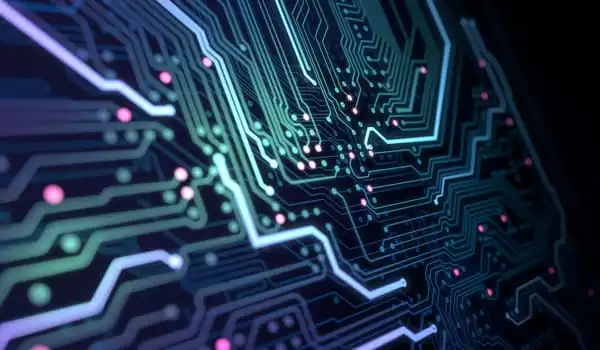
These events occur at incredibly fast rates, on the order of atto- or femtoseconds. “For a long time, such processes were thought to be instantaneous,” explains Prof. Christoph Lemell (TU Wien). “Today, however, we have the required capability to analyze the time evolution of these ultrafast processes in depth.” The essential question is how quickly the material reacts to the laser. How long does it take to generate a signal, and how long must one wait before the material may be exposed to the next signal? The experiments were carried out at Garching and Graz, while the theoretical study and complex computer simulations were carried out in TU Wien.
Time or energy — but not both
The experiment creates a classic uncertainty paradox, as is common in quantum physics: in order to enhance the speed, extremely brief UV laser pulses are required, allowing free charge carriers to be produced very quickly. The use of extremely short pulses, on the other hand, implies that the quantity of energy delivered to the electrons is not exactly defined. Electrons may absorb a wide range of energy. “We can detect when the free charge carriers are formed, but not what energy state they are in,” says Christoph Lemell. “Solids have different energy bands, and several of them are necessarily occupied by free charge carriers at the same time with brief laser pulses.”
The electrons react to the electric field differently depending on how much energy they carry. If their exact energy is unknown, they can no longer be accurately controlled, and the current signal produced is distorted – especially at high laser intensities.
According to Joachim Burgdörfer, “it turns out that roughly one petahertz is an upper limit for regulated optoelectronic operations.” Of course, this does not imply that computer chips with clock frequencies slightly below one petahertz are feasible. Realistic technical upper bounds are almost certainly much lower. Although the rules of nature that govern the ultimate speed limits of optoelectronics cannot be circumvented, they can now be analyzed and comprehended using sophisticated new approaches.