Physicists from Ludwig-Maximilian University in Munich (LMU) and the Max Planck Institute for Quantum Optics (MPQ) investigated the dynamics of photoelectron emission in tungsten crystals using ultrashort laser pulses.
Albert Einstein was awarded the Nobel Prize in Physics almost a century ago for his explanation of the photoelectric effect. Einstein’s theory, published in 1905, included the idea that light is made up of particles known as photons. When light strikes matter, the electrons in the sample react to the energy input, resulting in what is known as the photoelectric effect. The material absorbs light quanta (photons) and excites the bound electrons. This can result in electron ejection depending on the wavelength of the light source. The electronic band structure of the material involved has a significant impact on photoemission timescales.
Physicists from Ludwig-Maximilian University (LMU) in Munich and the Max Planck Institute for Quantum Optics (MPQ) have now studied the phenomenon of photoemission in greater depth. They measured the effect of tungsten band structure on the dynamics of photoelectron emission and provide theoretical explanations for their findings.
Physicists have used ultrashort laser pulses to probe the dynamics of photoelectron emission in tungsten crystals.
This is now possible as a result of the advancement and ongoing refinement of attosecond technology. The ability to generate trains of laser light pulses that last a few hundred attoseconds allows researchers to follow the course of photoemission by ‘freezing the action’ at regular intervals, similar to a stroboscope, but with far better temporal resolution.
The researchers used attosecond pulses of extreme ultraviolet light to investigate the dynamics of photoemission from a tungsten crystal in a series of photoelectron spectroscopy experiments. Each pulse contained several hundred X-ray photons, each of which was powerful enough to dislodge a photoelectron. The team was able to characterize the ejected electrons in terms of their times of flight and angles of emission using detectors mounted in front of the crystal.
The findings revealed that electrons that interact with incoming photons take a little time to react. This discovery was made possible by the use of a novel method for producing attosecond pulses. The addition of a passive cavity resonator with an enhancement factor of 35 allows the new setup to generate attosecond pulses at a rate of 18.4 million per second, which is approximately 1000-fold faster than that previously seen in comparable systems. Because of the high pulse repetition rate, only a few photoelectrons per pulse are required to provide a high average flux.
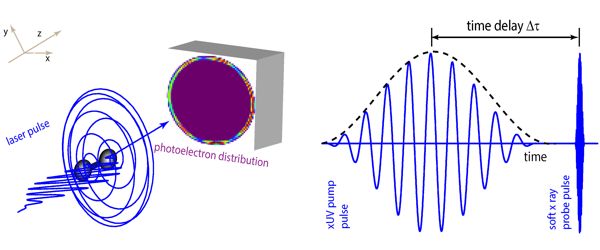
“Because negatively charged photoelectrons repel one another, their kinetic energies change rapidly. It is therefore critical to distribute them over as many attosecond pulses as possible in order to characterize their dynamics “Dr. Tobias Saule, co-first author, explains. Because the particles are well distributed in time and space due to the increased pulse rate, they have little opportunity to interact with each other, allowing the maximal energy resolution to be preserved.
The team was able to demonstrate that, in terms of photoemission kinetics, electrons in neighboring energy states in the valence band (i.e. the outermost orbits of the atoms in the crystal) with different angular momenta also differ by a few tens of attoseconds in the time it takes to respond to incoming photons.
Notably, the arrangement of the atoms within the crystal has a measurable effect on the delay between the arrival of the light pulse and the ejection of photoelectrons. “A crystal is made up of many atoms, all of which have positively charged nuclei.” “In the same way that a round hole acts as a potential well for marbles, each nucleus is the source of an electrical potential that attracts negatively charged electrons,” says Dr. Stephan Heinrich, who is also a co-first author on the report. “When an electron is dislodged from a crystal, what happens is similar to the movement of a marble across a pitted table with depressions.
These indentations represent the positions of the individual atoms in the crystal and are organized in a regular pattern. “Their presence has a direct effect on the trajectory of the marble, and it differs from what would be observed on a smooth surface,” he explains. “We’ve now shown how such a periodic potential within a crystal affects the temporal behavior of photoemission – and we can theoretically account for it,” Stephan Heinrich says. The observed delays can be attributed to the complex nature of electron transport from the interior to the surface of the crystal, as well as the impact of electron scattering and correlation effects.
“Our findings pave the way for experimental investigations of the complex interactions that occur in multi-electron systems in condensed matter on an attosecond timescale. This will allow us to theoretically understand them “Prof. Ulf Kleineberg of LMU, who led the project, says
Longer term, the new findings could lead to novel materials with electronic properties that improve light-matter interactions, making solar cells more efficient, and improving switching rates of nano-optical components for ultrafast data processing, as well as promoting the development of nanosystems for use in biomedical sciences.