The Moon no longer has a magnetic field, and models of its core suggest that it was likely too small and lacked the convective force to ever produce a continuously strong magnetic field. A core must dissipate a lot of heat in order to have a strong convective churn.
A new study explains how the Moon could have been an occasional magnetic powerhouse early in its history, a question that has baffled scientists since NASA’s Apollo program began in the 1960s.
Returning rocks to Earth during NASA’s Apollo program from 1968 to 1972 provided volumes of information about the Moon’s history, but they also sparked an ongoing mystery. The rocks’ analysis revealed that some appeared to have formed in the presence of a strong magnetic field, one that rivaled Earth’s in strength. However, it was unclear how a body the size of the Moon could have generated such a powerful magnetic field.
A new study led by a Brown University geoscientist proposes a new explanation for the Moon’s magnetic mystery. The study, published in Nature Astronomy, demonstrates that giant rock formations sinking through the Moon’s mantle could have produced the type of interior convection that generates strong magnetic fields. The processes could have produced intermittently strong magnetic fields for the first billion years of the Moon’s history, the researchers say.
“Everything we’ve thought about how magnetic fields are generated by planetary cores tells us that a body the size of the Moon should not be able to generate a field as strong as Earth’s,” said Alexander Evans, an assistant professor of Earth, environmental, and planetary sciences at Brown and co-author of the study with Stanford University’s Sonia Tikoo. “Instead of pondering how to sustain a strong magnetic field over billions of years, perhaps there is a way to generate a high-intensity field intermittently. Our model explains how that could happen, and it is consistent with what we know about the interior of the Moon.”
This model is able to explain both the intensity and the variability we see in the Apollo samples – something that no other model has been able to do. It also gives us some time constraints on the foundering of this titanium material, which gives us a better picture of the Moon’s early evolution.
Alexander Evans
Planetary bodies produce magnetic fields through what’s known as a core dynamo. Slowly dissipating heat causes convection of molten metals in a planet’s core. The constant churning of electrically conductive material is what produces a magnetic field. That’s how Earth’s magnetic field — which protects the surface from the sun’s most dangerous radiation — is formed.
The Moon no longer has a magnetic field, and models of its core suggest that it was likely too small and lacked the convective force to ever produce a continuously strong magnetic field. A core must dissipate a lot of heat in order to have a strong convective churn. Evans claims that in the case of the early Moon, the mantle surrounding the core was not much cooler than the core itself. There was little convection in the core because the heat in the core had nowhere to go. This new study, however, demonstrates how sinking rocks could have provided intermittent convective boosts.
The story of these sinking stones begins a few million years after the formation of the Moon. The Moon is thought to have been covered by an ocean of molten rock very early in its history. As the vast magma ocean began to cool and solidify, denser minerals like olivine and pyroxene sank to the bottom, while less dense minerals like anorthosite floated to form the crust.
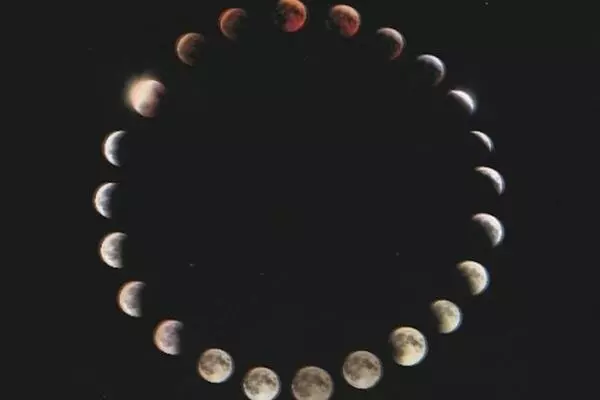
Because the remaining liquid magma contained a high concentration of titanium as well as heat-producing elements such as thorium, uranium, and potassium, it took a little longer to solidify. When this titanium layer finally crystallized just beneath the crust, it was denser than the minerals that had previously solidified beneath it. Over time, the titanium formations sank through the less-dense mantle rock underneath, a process known as gravitational overturn.
Evans and Tikoo modeled the dynamics of how those titanium formations would have sunk, as well as the effect they might have had when they eventually reached the Moon’s core, for this new study. Based on the Moon’s current composition and estimated mantle viscosity, the formations would most likely break into blobs as small as 60 kilometers in diameter and sink intermittently over the course of a billion years.
The researchers discovered that when each of these blobs eventually hit bottom, they would have delivered a significant shock to the Moon’s core dynamo. The titanium formations would have been relatively cool in temperature, having been perched just beneath the Moon’s crust – far cooler than the core’s estimated temperature of between 2,600 and 3,800 degrees Fahrenheit. When the cool blobs collided with the hot core after sinking, the temperature difference would have increased core convection, resulting in a magnetic field at the Moon’s surface that was as strong as, if not stronger than, Earth’s.
“Think of it like a drop of water hitting a hot skillet,” Evans explained. “When something extremely cold comes into contact with the core, a large amount of heat can suddenly escape. This increases churning in the core, resulting in these intermittently strong magnetic fields.”
According to the researchers, there could have been up to 100 of these downwelling events during the Moon’s first billion years of existence, each of which could have produced a strong magnetic field lasting a century or so.
Evans says the intermittent magnetic model not only accounts for the strength of the magnetic signature found in the Apollo rock samples, but also for the fact that magnetic signatures vary widely in the Apollo collection — with some having strong magnetic signatures while others don’t.
“This model is able to explain both the intensity and the variability we see in the Apollo samples — something that no other model has been able to do,” Evans said. “It also gives us some time constraints on the foundering of this titanium material, which gives us a better picture of the Moon’s early evolution.”
Evans claims that the concept is also quite testable. It implies that the Moon had a weak magnetic background that was punctuated by these high-strength events. That should be clear from the Apollo collection. While the strong magnetic signatures in the Apollo samples stood out like a sore thumb, Evans claims that no one has ever looked for weaker signatures. The presence of those weak signatures alongside the strong ones would give this new idea a big boost, potentially putting an end to the Moon’s magnetic mystery.