The physics of our universe is a vast and complex subject that involves the study of the fundamental laws of nature and the behavior of matter and energy at all scales, from the subatomic level to the largest structures in the cosmos. To better understand the physics of our universe, scientists use a variety of experimental techniques, mathematical models, and advanced technologies to study and observe the natural world.
Researchers from all over the world have worked to find answers to fundamental physics questions that govern our universe. The Majorana Demonstrator, their experiment, has helped to broaden the scope of research into neutrinos, one of the fundamental building blocks of the universe.
For the past six years, Indiana University researchers and collaborators from all over the world have worked to answer fundamental questions about the physical laws that govern our universe. The Majorana Demonstrator, their experiment, has helped to broaden the scope of research into neutrinos, one of the fundamental building blocks of the universe.
The experiment’s final report was published in Physical Review Letters.
Neutrinos have a profound impact on the universe and physics at every imaginable scale, surprising us down at the particle interaction level and having a broad impact up through the cosmic scales.
Walter Pettus
Neutrinos, which are subatomic particles similar to electrons but lack an electric charge, are the universe’s second most abundant particle after light. They are, however, some of the most difficult particles to measure because they do not interact in the same way that other particles do.
“Neutrinos have a profound impact on the universe and physics at every imaginable scale, surprising us down at the particle interaction level and having a broad impact up through the cosmic scales,” said Walter Pettus, an assistant professor of physics in the Indiana University College of Arts and Sciences. “However, they are also the most difficult to study because we know so much about them but have so many gaps.”
The Majorana Demonstrator, a collaboration of 60 researchers from 24 institutions, was designed to fill many of those gaps at the same time, probing into the most fundamental properties of neutrinos.
One aspect they hoped to investigate was whether the neutrino could be its own antiparticle – a subatomic particle with the same mass but the opposite electric charge. Because the neutrino is uncharged, it is the only particle in the universe that can be its own antiparticle. Understanding this would provide insight into why the neutrino has mass in the first place, which would have far-reaching implications for understanding how the universe was formed.
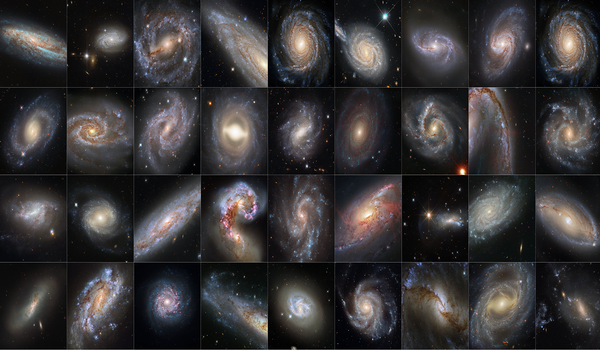
To determine if the neutrino is its own antiparticle, the researchers needed to observe a rare occurrence called neutrinoless double-beta decay. However, this process takes a single atom at least 1026 years — significantly longer than the age of the universe. Instead, they chose to observe nearly 1026 atoms over the course of six years.
The researchers needed the ideal conditions to observe this extremely rare decay. They created one of the cleanest and quietest environments on Earth at the Sanford Underground Research Facility in the Black Hills of South Dakota, which is a mile underground. Extremely sensitive germanium detectors were packed in a 50-ton lead shield and surrounded by materials of unprecedented cleanliness. Even the copper used in their lab was grown underground with impurity levels so low that they couldn’t be measured.
Pettus and a team of IU students were responsible primarily for analyzing data from the experiment. Graduate student Nafis Fuad, undergraduate senior Isaac Baker, sophomore Abby Kickbush and Jennifer James, a student with the Research Experiences for Undergraduates Program, have been involved in the project. Their focus has been on understanding the stability of the experiment, analyzing details of the recorded waveforms and characterizing backgrounds.
“It’s like looking for a tiny needle in a very, very, very big haystack — you have to carefully get rid of all the hays (a.k.a. backgrounds) possible, and you don’t even know if there’s a needle in there in the first place,” Fuad explained. “It’s a thrill to be a part of that hunt.”
While the researchers did not observe the desired decay, they did discover that the neutrino’s decay scale is longer than the limit they set, which they will test further during the next phase of the experiment. In addition, they recorded other scientific results ranging from dark matter to quantum mechanics that help provide a better understanding of the universe.
The researchers demonstrated that the techniques they used could be used on a much larger scale in a potentially game-changing search to explain the existence of matter in the universe.
“We didn’t find the decay we were looking for, but we raised the bar on where to look for the physics we’re looking for,” Pettus explained. “The Demonstrator, true to its name, advanced critical technologies that we are already leveraging for the next phase of the experiment in Italy.” We may not have broken the physics picture yet, but we have certainly pushed the horizons, and I am very proud of what we have accomplished.”
The project’s next phase, known as LEGEND-200, has already begun collecting data in Italy, with plans to continue for the next five years. The researchers hope to observe the decay with a magnitude greater sensitivity than the Majorana Demonstrator. In addition, with funding from the US Department of Energy, the team is already planning the successor experiment, LEGEND-1000.
Pettus is excited about the future of this work and hopes to involve more students in data analysis and hardware development for the LEGEND-1000.