Researchers have made a breakthrough that should pave the way for much better control over the formation of quantum bits, or qubits, the fundamental unit of quantum information technology. The findings of the team could aid in the development of industrially relevant quantum materials for sensing, computing, and communication.
When looking for a hotel room on a road trip, “Vacancy” is a good sign to look for. When it comes to quantum materials, vacancies are also desirable. They are created by scientists by removing atoms from crystalline materials. Such vacancies can be used to create quantum bits, or qubits, the fundamental unit of quantum technology.
Researchers at the Department of Energy’s (DOE) Argonne National Laboratory and the University of Chicago have made a breakthrough that should pave the way for much better control over the formation of vacancies in silicon carbide, a semiconductor.
Semiconductors are the brains of cell phones, computers, medical equipment, and other electronic devices. The presence of atomic-scale defects in the form of vacancies is undesirable for those applications because they can interfere with performance. Recent research, however, suggests that certain types of vacancies in silicon carbide and other semiconductors hold promise for the realization of qubits in quantum devices. Qubits could be used to create unhackable communication networks and hypersensitive sensors capable of detecting individual molecules or cells. New types of computers capable of solving complex problems beyond the capabilities of traditional computers are also possible in the future.
We can watch defects forming, moving, disappearing, and rotating in a sample over time at different temperatures by performing computer simulations at the atomic scale with high-performance computers. At the moment, this is something that cannot be done experimentally.
Elizabeth Lee
“Scientists already know how to create qubit-worthy vacancies in semiconductors like silicon carbide and diamond,” said Giulia Galli, a senior scientist at Argonne’s Materials Science Division and a University of Chicago professor of molecular engineering and chemistry. “However, in order to develop practical new quantum applications, they will need to learn a lot more about how to customize these vacancies with desired features.”
Single vacancies form in silicon carbide semiconductors when individual silicon and carbon atoms are removed from the crystal lattice. A carbon vacancy, for example, can pair with an adjacent silicon vacancy. This paired vacancy, known as a divacancy, is a promising candidate for a silicon carbide qubit. The issue has been that the yield for converting single vacancies into divacancies has been low, only a few percentage points. Scientists are working feverishly to find a way to boost that yield.
“To create actual defects in a sample, you shoot a beam of high-velocity electrons at it, and this knocks out individual atoms,” Elizabeth Lee, a postdoctoral researcher at the University of Chicago Pritzker School of Molecular Engineering, explained. ? “However, that electron bombardment creates unwanted defects.”
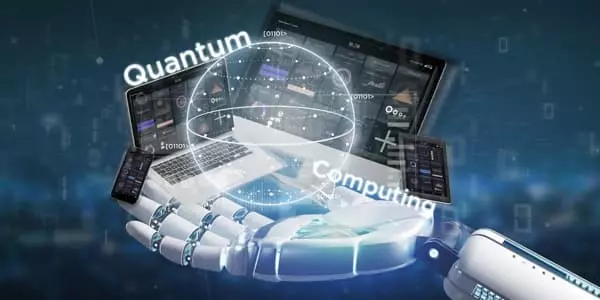
Scientists can repair those flaws by heating the sample to temperatures above 1,300 degrees Fahrenheit and then cooling it back down to room temperature. The trick is to create a process that keeps the desired defects while healing the unwanted ones.
“We can watch defects forming, moving, disappearing, and rotating in a sample over time at different temperatures by performing computer simulations at the atomic scale with high-performance computers,” Lee explained. “At the moment, this is something that cannot be done experimentally.”
The team’s simulations, which were aided by a combination of sophisticated computational tools, tracked the pairing of individual vacancies into a divacancy. Their efforts bore fruit in the form of seminal discoveries that should pave the way for new quantum devices. The first is that the more silicon vacancies there are relative to carbon vacancies at the start of heat treatment, the more divacancies there will be later. Another challenge is determining the best temperatures for creating stable divacancies and reorienting them within the crystal structure without destroying them.
Scientists may be able to use the latter discovery to align all divacancies in the same direction. That would be highly desirable for sensing applications capable of operating with many orders of magnitude higher resolution than today’s sensors.
“A completely unexpected and exciting discovery was that divacancies can transform into an entirely new type of defect,” Lee added. These newly discovered defects are made up of two carbon vacancies joined by an anti-site, as scientists call it. That is a location where a carbon atom has filled the void left by the removal of a silicon atom.
The team’s simulations were the first of their kind, thanks to the development of new simulation algorithms and the coupling of computer codes developed by the DOE-funded Midwest Integrated Center for Computational Materials (MICCoM), which is headquartered at Argonne and led by Galli. The new algorithms were developed by Juan de Pablo, a senior scientist in the Materials Science Division and a UChicago professor of molecular engineering. They are based on concepts from machine learning, a type of artificial intelligence.
“The formation and motion of vacancies or defects in semiconductors are rare events,” de Pablo explained. “Such events occur on time scales that are far too long to study using conventional molecular simulations, even on the world’s fastest computer. It is critical that we find new ways to encourage the occurrence of these events without changing the underlying physics. Our algorithms do exactly that; they make the impossible possible.”
Lee connected the various codes, building on the work of MICCoM researchers Galli and de Pablo. Several other scientists, including Francois Gygi at the University of California, Davis, and Jonathan Whitmer at Notre Dame University, have been involved in code coupling over the years. The result is a significant and powerful new toolkit for investigating vacancy formation and behavior that combines quantum theory and simulations. This will apply not only to silicon carbide, but also to other promising quantum materials.
“We’re just getting started,” Galli said. “We want to be able to compute much faster, simulate many more defects, and determine which defects are best for different applications.”