Using machine learning and simulations of massive impacts, researchers discovered that the planets in the inner solar system were most likely formed by repeated hit-and-run collisions, challenging conventional models of planet formation.
According to new research led by researchers at the University of Arizona Lunar and Planetary Laboratory, planet formation – the process by which neat, round, distinct planets form from a roiling, swirling cloud of rugged asteroids and mini planets – was likely messier and more complicated than most scientists would care to admit.
The findings contradict the widely held belief that collisions between smaller building blocks cause them to stick together and, over time, repeated collisions add new material to the growing baby planet.
Instead, the authors propose and demonstrate evidence for a novel “hit-and-run-return” scenario in which pre-planetary bodies spent a significant portion of their journey through the inner solar system crashing into and ricocheting off of each other, before colliding again later. They would be more likely to stick together the next time because their first collision had slowed them down. Imagine a game of billiards with the balls coming to rest, rather than pelting a snowman with snowballs.
The findings are detailed in two reports published in the September 23 issue of The Planetary Science Journal, one focusing on Venus and Earth and the other on Earth’s moon. According to the author team, which was led by planetary sciences and LPL professor Erik Asphaug, the largely unrecognized point that giant impacts are not the efficient mergers scientists thought they were is central to both publications.
In our opinion, Earth would have accreted most of its material from head-on collisions, or else at a slower rate than Venus. More oblique and higher velocity collisions into the Earth would have preferredentially ended up on Venus.
Professor Erik Asphaug
“We discovered that the majority of giant impacts, even relatively ‘slow’ ones, are hit-and-runs.” This means that in order for two planets to merge, they must first slow down in a hit-and-run collision,” Asphaug explained. “Thinking of giant impacts, such as the formation of the moon, as a single event is probably incorrect. More likely it took two collisions in a row.”
One implication is that Venus and Earth would have had very different experiences in their growth as planets, despite being immediate neighbors in the inner solar system. In the first paper, led by Alexandre Emsenhuber, who did this work during a postdoctoral fellowship in Asphaug’s lab and is now at Ludwig Maximilian University in Munich, the young Earth would have served to slow down interloping planetary bodies, making them ultimately more likely to collide with and stick to Venus.
“We think that during solar system formation, the early Earth acted like a vanguard for Venus,” Emsenhuber said.
The solar system is a gravity well, which is the concept behind a popular attraction at science museums. Visitors toss a coin into a funnel-shaped gravity well, then watch as their money orbits several times before falling into the center hole. Planets experience greater gravitational attraction as they get closer to the sun. That’s why the inner planets of the solar system studied here, Mercury, Venus, Earth, and Mars, orbit the sun faster than Jupiter, Saturn, and Neptune. As a result, the closer an object approaches the sun, the more likely it will remain there.
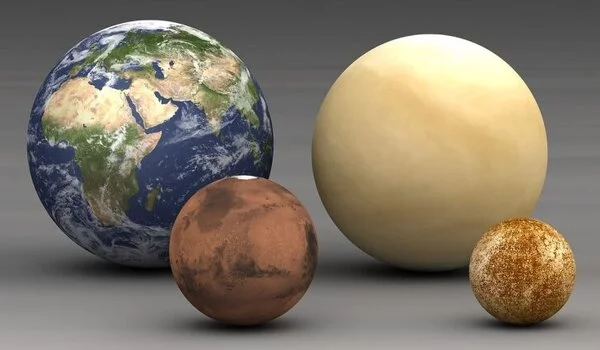
As a result, Asphaug explained, when an interloping planet collided with Earth, it was less likely to stick to Earth and more likely to end up at Venus. “The Earth acts as a shield, providing a first line of defense against these incoming planets,” he explained. “A planet that bounces off of Earth is more likely to collide with Venus and merge with it.”
Emsenhuber illustrates what drives the vanguard effect with the analogy of a ball bouncing down a staircase: A body entering the solar system is analogous to a ball bouncing down a flight of stairs, with each bounce representing a collision with another body.
“Along the way, the ball loses energy, and you’ll find it will always bounce downstairs, never upstairs,” he said. “Because of that, the body cannot leave the inner solar system anymore. You generally only go downstairs, toward Venus, and an impactor that collides with Venus is pretty happy staying in the inner solar system, so at some point it is going to hit Venus again.”
Earth has no such vanguard to slow down its interloping planets. This leads to a difference between the two similar-sized planets that conventional theories cannot explain, the authors argue.
“The prevailing idea has been that it doesn’t really matter if planets collide and don’t merge right away, because they are going to run into each other again at some point and merge then,” Emsenhuber said. “But that is not what we find. We find they end up more frequently becoming part of Venus, instead of returning back to Earth. It’s easier to go from Earth to Venus than the other way around.”
The team used machine learning to obtain predictive models from 3D simulations of giant impacts in order to track all of these planetary orbits and collisions, and eventually their mergers. The team then used this information to rapidly compute the orbital evolution, including hit-and-run and merging collisions, in order to simulate terrestrial planet formation over a 100-million-year time span. The authors propose and demonstrate their hit-and-run-return scenario for the moon’s formation in the second paper, recognizing the primary problems with the standard giant impact model.
“The standard model for the moon requires a relatively slow collision, and it creates a moon that is mostly composed of the impacting planet, not the proto-Earth, which is a major problem because the moon has an isotopic chemistry almost identical to Earth,” Asphaug explained.
In the team’s new scenario, a protoplanet roughly the size of Mars collides with Earth, as in the standard model, but is a little faster, allowing it to keep going. It returns in about 1 million years with a massive impact that resembles the standard model.
“The double impact mixes things up much more than a single event,” Asphaug said, “which could explain the isotopic similarity of Earth and moon, and also how the second, slow, merging collision would have happened in the first place.”
The researchers believe that the resulting asymmetry in how the planets were assembled points the way to future research on the diversity of terrestrial planets. For example, we don’t know how Earth got its much stronger magnetic field than Venus, or why Venus doesn’t have a moon. According to Asphaug, their research reveals systematic differences in dynamics and composition.
“In our opinion, Earth would have accreted most of its material from head-on collisions, or else at a slower rate than Venus,” he said. “More oblique and higher velocity collisions into the Earth would have preferredentially ended up on Venus.”