Quantum fluctuations of the vacuum are a well-established concept in quantum field theory, and they play an important role in our understanding of particle physics. However, it is important to note that these fluctuations are typically not directly observable in the traditional sense. They are mathematical descriptions used to explain certain phenomena in quantum mechanics and quantum field theory.
Most of us imagine the vacuum as completely empty. Yet, in reality, it is filled with an energetic flickering: quantum fluctuations. Experts are currently preparing a laser experiment to verify these vacuum fluctuations in a novel way, which could potentially provide clues to new physics laws. A research team from the Helmholtz-Zentrum Dresden-Rossendorf (HZDR) has developed a series of proposals designed to help conduct the experiment more effectively – thus increasing the chances of success. The team presents its findings in the scientific journal Physical Review D.
The physics community has long known that the vacuum is not completely empty, but rather filled with vacuum fluctuations, an ominous quantum flickering in time and space. Although it cannot be directly observed, its influence can be observed indirectly, for example, by changes in the electromagnetic fields of tiny particles.
However, it has not yet been possible to demonstrate vacuum fluctuations in the absence of particles. If this is accomplished, one of physics’ fundamental theories, quantum electrodynamics (QED), will be proven in a previously untested area. However, if such an experiment reveals deviations from the theory, it would imply the existence of new, previously unknown particles.
It would be like sliding a transparent plastic ruler between two polarizing filters and bending it back and forth. The filters are originally set up so that no light passes through them. Bending the ruler would now change the direction of the light’s oscillation in such a way that something could be seen as a result.
Prof. Ralf Schützhold
The experiment is part of the Helmholtz International Beamline for Extreme Fields (HIBEF), a research consortium led by the HZDR at the HED experimental station of the European XFEL in Hamburg, the world’s largest X-ray laser. The underlying principle is that an ultra-powerful laser fires short, intense flashes of light into a stainless steel chamber that has been evacuated. The goal is to manipulate vacuum fluctuations in such a way that they appear to magically change the polarization of an X-ray flash from the European XFEL, i.e. rotate its direction of oscillation.
“It would be like sliding a transparent plastic ruler between two polarizing filters and bending it back and forth,” explains HZDR theorist Prof. Ralf Schützhold. “The filters are originally set up so that no light passes through them. Bending the ruler would now change the direction of the light’s oscillation in such a way that something could be seen as a result.” In this analogy, the ruler corresponds to the vacuum fluctuations while the ultra-powerful laser flash bends them.
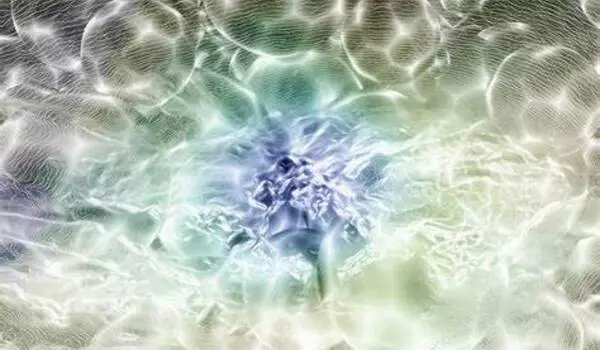
Two flashes instead of just one
The original idea was to fire a single optical laser flash into the chamber and then use specialized measurement techniques to see if it changed the polarization of the X-ray flash. But there’s a catch: “The signal is likely to be extremely weak,” Schützhold says. “It is possible that only one in a trillion X-ray photons will change its polarization.”
However, this may be below the current measurement limit, allowing the event to slip through the cracks unnoticed. As a result, Schützhold and his colleagues are relying on a variant: instead of just one, they intend to fire two optical laser pulses into the evacuated chamber at the same time.
Both flashes will strike there and literally collide. The X-ray pulse of the European XFEL is set to fire precisely into their collision point. The decisive factor: The colliding laser flashes affect the X-ray pulse like a type of crystal. Just as X-rays are diffracted, i.e., deflected, when passing through a natural crystal, the XFEL X-ray pulse should also be deflected by the briefly existing “light crystal” of the two colliding laser flashes.
“That would not only change the polarization of the X-ray pulse but also slightly deflect it at the same time,” explains Ralf Schützhold. This combination could increase the chances of actually being able to measure the effect – so the researchers hope. The team has calculated various options for the striking angle of the two laser flashes colliding in the chamber. Experiments will show which variant proves to be most suitable.
Targeting ultra-light ghost particles?
The prospects could be improved even further if the two laser flashes fired into the chamber were of different wavelengths rather than the same color. This would also allow the energy of the X-ray flash to change slightly, aiding in the measurement of the effect. “But this is technically quite challenging and may only be implemented at a later date,” Schützhold said.
The project is currently being planned in Hamburg with the European XFEL team at the HED experimental station, with the first trials set to begin in 2024. If they are successful, they may be able to confirm QED once more.
But perhaps the experiments will reveal deviations from the established theory. This could be due to previously undiscovered particles – for example, ultra-light ghost particles known as axions. “And that,” says Schützhold, “would be a clear indication of additional, previously unknown laws of nature.”