Electrochemistry is the study of the relationship between electricity and chemical reactions. It can be used to convert carbon-based feedstocks, such as coal or natural gas, into useful molecules like fuels, chemicals, and materials. This is typically done through the use of electrolysis, which involves passing an electric current through a solution or a molten material to produce a chemical reaction.
For example, electrolysis can be used to produce hydrogen gas from water by splitting the water molecule into its constituent hydrogen and oxygen atoms. This process is known as water electrolysis and is often used to produce hydrogen fuel for use in fuel cells or as a chemical feedstock. Electrolysis can also be used to produce methanol, a chemical intermediate that can be used to make a wide variety of products including plastics, paints, and pharmaceuticals.
A chemistry collaboration resulted in a novel way to put carbon dioxide to good – and even healthy – use: by electrosynthesising it into a series of organic molecules critical to pharmaceutical development. During the process, the team made a novel discovery. They could produce two completely different products, both of which are useful in medicinal chemistry, by changing the type of electrochemical reactor.
The team’s paper, “Electrochemical Reactor Dictates Site Selectivity in N-Heteroarene Carboxylations,” published in Nature. The paper’s co-lead authors are postdoctoral researchers Peng Yu and Wen Zhang, and Guo-Quan Sun of Sichuan University in China.
This is the first time we discovered that changing the cell, or what we call the electrochemical reactor, completely changes the product. I believe that gaining a mechanistic understanding of what happened will enable us to apply the same strategy to other molecules, not just pyridines, and possibly make other molecules in this selective but controlled manner.
Prof. Song Lin
The Cornell team, led by Song Lin, professor of chemistry and chemical biology in the College of Arts and Sciences, has previously used electrochemistry to stitch together simple carbon molecules and form complex compounds, eliminating the need for precious metals or other catalysts to promote the chemical reaction.
They focused on pyridine, the second-most common heterocycle in FDA-approved drugs, for the new project. Heterocyles are organic compounds in which the atoms of the molecules are linked into ring structures, at least one of which is not carbon. These structural units are referred to as “pharmacophores” due to their frequent presence in medicinally active compounds. They are also commonly found in agrochemicals.
The researchers’ goal was to make carboxylated pyridines, i.e., pyridines with carbon dioxide appended to them. The advantage of introducing carbon dioxide to a pyridine ring is that it can change a molecule’s functionality and potentially help it bind to certain targets, such as proteins. However, the two molecules are not natural partners. Pyridine is a reactive molecule, while carbon dioxide is generally inert.
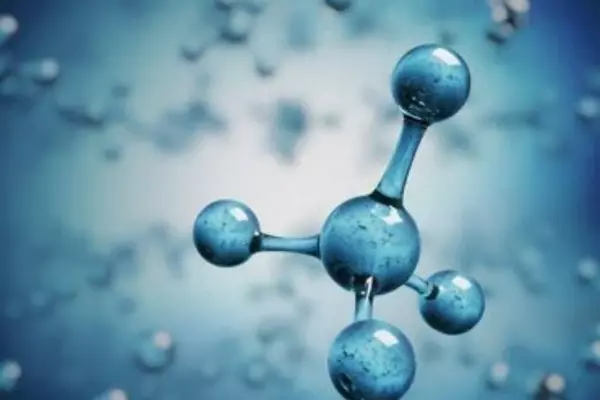
“There are very few ways of directly introducing carbon dioxide to a pyridine,” said Lin, the paper’s co-senior author, along with Da-Gang Yu of Sichuan University. “The current methods have very severe limitations.”
Lin’s lab combined its expertise in electrochemistry with Yu’s group’s specialization in utilizing carbon dioxide in organic synthesis, and they were able to successfully create carboxylated pyridines.
“Electrochemistry provides the leverage to dial in the potential that is sufficient to activate even the most inert molecules,” Lin explained. “This is how we got this reaction.”
During the electrosynthesis, the team made a chance discovery. Chemists typically conduct electrochemical reactions in one of two ways: in an undivided electrochemical cell (where the anode and cathode that supply the electric current are in the same solution) or in a divided electrochemical cell (whereby the anode and cathode are separated by a porous divider that blocks large organic molecules but allows ions to pass through). Although one method is more efficient than the other, they both produce the same result.
Lin’s group found that by switching from a divided to an undivided cell they could selectively attach the carbon dioxide molecule on different positions of the pyridine ring, creating two different products: C4-carboxylation in the undivided cell and C5-carboxylation in the divided cell.
“This is the first time we discovered that changing the cell, or what we call the electrochemical reactor, completely changes the product,” Lin explained. “I believe that gaining a mechanistic understanding of what happened will enable us to apply the same strategy to other molecules, not just pyridines, and possibly make other molecules in this selective but controlled manner. That, I believe, is a general principle that can be applied to other systems.”
While the project’s method of utilizing carbon dioxide will not solve the global problem of climate change, Lin believes it is “a small step toward using excess carbon dioxide in a useful way.”