Arrays of quantum rods have the potential to improve various display technologies, such as televisions and virtual reality (VR) devices. Quantum rods are nanoscale semiconductor structures that, when excited by an external energy source, emit light of specific colors.
Engineers assembled arrays of quantum rods with desirable photonic properties using folded DNA scaffolds, which could allow them to be used as highly efficient micro-LEDs for televisions or virtual reality devices.
Flat-screen TVs with quantum dots are now commercially available, but creating arrays of their elongated cousins, quantum rods, for commercial devices has been more difficult. Quantum rods can control both the polarization and color of light, allowing them to create 3D images for virtual reality devices.
MIT engineers have developed a new method for precisely assembling arrays of quantum rods by using scaffolds made of folded DNA. The researchers were able to control the polarization of light emitted by the array by depositing quantum rods onto a DNA scaffold in a highly controlled manner. This makes it easier to add depth and dimensionality to a virtual scene.
The quantum rods sit on the origami in the same direction, so now you have patterned all these quantum rods through self-assembly on 2D surfaces, and you can do that over the micron scale needed for different applications like microLEDs.
Mark Bathe
“One of the challenges with quantum rods is: How do you align them all at the nanoscale so they’re all pointing in the same direction?” says Mark Bathe, an MIT professor of biological engineering and the senior author of the new study. “When they’re all pointing in the same direction on a 2D surface, then they all have the same properties of how they interact with light and control its polarization.”
MIT postdocs Chi Chen and Xin Luo are the lead authors of the paper, which appears today in Science Advances. Robert Macfarlane, an associate professor of materials science and engineering; Alexander Kaplan PhD ’23; and Moungi Bawendi, the Lester Wolfe Professor of Chemistry, are also authors of the study.
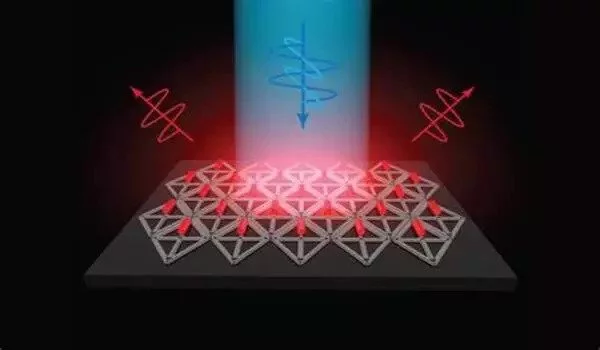
Nanoscale structures
Bathe and others have led the way in the design and fabrication of nanoscale DNA structures, also known as DNA origami, over the last 15 years. DNA, a highly stable and programmable molecule, is an excellent building material for tiny structures that could be used for a variety of purposes, such as drug delivery, biosensors, or scaffolds for light-harvesting materials.
Bathe’s lab has developed computational methods that allow researchers to enter a target nanoscale shape they want to create and the program will calculate the DNA sequences that will self-assemble into the desired shape. They also created scalable methods for incorporating quantum dots into these DNA-based materials.
Bathe and Chen demonstrated in a 2022 paper that they could use DNA to scaffold quantum dots in precise positions using scalable biological fabrication. Building on that work, they collaborated with Macfarlane’s lab to tackle the more difficult task of arranging quantum rods into 2D arrays, which is more difficult because the rods must be aligned in the same direction.
Existing approaches that use mechanical rubbing with a fabric or an electric field to sweep the rods in one direction to create aligned arrays of quantum rods have had limited success. This is because high-efficiency light-emission requires the rods to be kept at least 10 nanometers apart so that they do not “quench,” or suppress, the light-emitting activity of their neighbors.
To accomplish this, the researchers devised a method of attaching quantum rods to diamond-shaped DNA origami structures, which can be built at the appropriate size to maintain that distance. These DNA structures are then attached to a surface and assembled like puzzle pieces.
“The quantum rods sit on the origami in the same direction, so now you have patterned all these quantum rods through self-assembly on 2D surfaces, and you can do that over the micron scale needed for different applications like microLEDs,” says Bathe. “You can orient them in specific directions that are controllable and keep them well-separated because the origamis are packed and naturally fit together, as puzzle pieces would.”
Assembling the puzzle
As the first step in getting this approach to work, the researchers had to come up with a way to attach DNA strands to the quantum rods. To do that, Chen developed a process that involves emulsifying DNA into a mixture with the quantum rods, then rapidly dehydrating the mixture, which allows the DNA molecules to form a dense layer on the surface of the rods.
This process takes only a few minutes, much faster than any existing method for attaching DNA to nanoscale particles, which may be key to enabling commercial applications.
“The unique aspect of this method lies in its near-universal applicability to any water-loving ligand with affinity to the nanoparticle surface, allowing them to be instantly pushed onto the surface of the nanoscale particles. By harnessing this method, we achieved a significant reduction in manufacturing time from several days to just a few minutes,” Chen says.
These DNA strands then act like Velcro, allowing quantum rods to adhere to a DNA origami template, forming a thin film that coats a silicate surface. This thin film of DNA is first formed by self-assembly, which involves connecting neighboring DNA templates with overhanging strands of DNA along their edges.
The researchers now hope to create wafer-scale surfaces with etched patterns, which will enable them to scale their design to device-scale arrangements of quantum rods for a variety of applications other than microLEDs and augmented reality/virtual reality.
“The method described in this paper is fantastic because it allows us to have good spatial and orientational control over how the quantum rods are positioned.” The next step will be to create more hierarchical arrays with programmed structure at many different length scales. “The ability to control the sizes, shapes, and placement of these quantum rod arrays opens the door to a wide range of different electronic applications,” says Macfarlane.
“DNA is particularly appealing as a manufacturing material because it can be produced biologically, which is both scalable and sustainable, in line with the emerging bioeconomy in the United States.” “What we’re focusing on next is translating this work into commercial devices by addressing several remaining bottlenecks, such as switching to environmentally safe quantum rods,” Bathe adds.