Scientists have demonstrated a broad range of tunability in a family of qubits, which is a key step toward building tailored qubits for specific uses. A novel foundation for developing diverse, highly customized quantum devices emerges from a ground-up approach to qubit design.
Quantum physics advancements have the potential to change the way we live. Quantum computers have the potential to solve problems that are currently unsolvable, and we may one day deploy quantum networks as hacker-proof information highways.
The development of such cutting-edge technology is heavily reliant on the qubit, the fundamental component of quantum systems. A significant difficulty in qubit research is engineering them to be configurable, to function with a wide range of sensing, communication, and computational devices.
Scientists have made significant progress in the development of customized qubits. The team, which includes researchers from MIT, the University of Chicago, and Columbia University, demonstrates how a specific molecular family of qubits can be finely tuned over a broad spectrum, similar to turning a sensitive dial on a wideband radio, in a paper published in the Journal of the American Chemical Society. The team also describes the fundamental design elements that allow for precise control of these quantum bits.
“This is a new framework for designing qubits. We can develop a novel quantum system using our predictable, controlled, and customizable design technique “Danna Freedman, an MIT chemistry professor and co-author of the paper, agreed. “We’ve demonstrated the great range of adaptability that these design concepts provide.”
Q-NEXT, a U.S. Department of Energy (DOE) National Quantum Information Science Research Center directed by Argonne National Laboratory, is funding a portion of the research. The study focuses on a specific type of molecule: those with a core chromium atom surrounded by four hydrocarbon molecules to form a pyramid-like shape.
This is a new framework for designing qubits. We can develop a novel quantum system using our predictable, controlled, and customizable design technique. We’ve demonstrated the great range of adaptability that these design concepts provide.
Professor Danna Freedman
The molecular qubit advantage
The qubit is the quantum counterpart to the classical computing bit. It can take numerous physical forms, such as a properly prepared atom inside a crystal or an electrical circuit. It could also be a molecule created in a laboratory. A molecular qubit has the advantage of being able to be created from the ground up, similar to a tiny 3D-printed gadget, providing the scientist the ability to adjust the qubit for varied functions.
“We’re working to change the atomic structure using synthetic chemistry and then studying how those changes modify the physics of the qubit,” said Leah Weiss, a postdoctoral researcher and study co-author at the University of Chicago.
The information in a molecular qubit is stored in its spin, which is an atomic-level material feature. Scientists engineer spin by modifying – tuning – the arrangement of the electrons in the molecule, known as its electronic structure. The information enters the qubit as light particles, or photons, and is encoded in the spin of the qubit. The spin-encoded data is then converted back into photons and read out.
Different photon wavelengths are better suited to certain purposes. One wavelength may be better suited for biosensing applications, while another may be better suited for quantum communication.
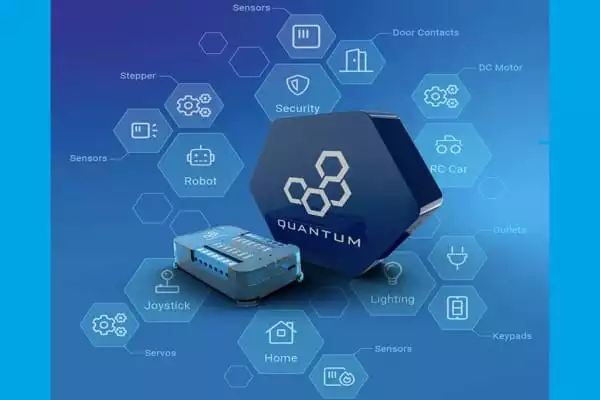
The ligand’s the thing
The ligand field strength, or the strength of the bonds connecting the central metal atom to the surrounding hydrocarbons, is one of the molecular qubit’s primary tuning dials. “Fundamentally, the ligand is everything. We can deliberately manage how the ligand environment changes the spin and logically control where the photons are emitted “said Dan Laorenza, an MIT graduate student and the paper’s principal author.
Researchers revealed that they could fine-tune these connections to an astonishing degree. They also demonstrated that the ligand field strengths are adjustable over a reasonably large range, while computational simulations done by Columbia researchers provided quantum mechanical insight into the ligands’ function in influencing the molecule’s electrical properties.
Their chromium qubits emitted light that stretched an astonishing 100 nanometers. “This is an unprecedented level of tunability for qubits aimed at designer applications,” added Freedman.
“You can play around with the properties just by keeping the central metal ion the same, which is doing the hard work of the quantum information processing, but tuning the surrounding environment through ligands,” said Sam Bayliss of the University of Glasgow, who co-authored the study while a postdoctoral researcher at the University of Chicago. “That’s really difficult to achieve in other systems, such as solid-state systems, where you’re effectively fixed at whatever the elemental properties provide you.”
A solid-state qubit is made by extracting a tiny, atom-sized bit of matter from a crystal, and the ensuing vacancy is where quantum information is stored and processed. While they offer advantages, solid-state qubits, for example, cannot be tweaked with the same chemical accuracy.
“With that, you practically get no adjustment,” Freedman explained. “You’re really going from zero to one hundred there.”
Laying out the design rules
Approaching the molecule’s design by focusing on its electronic structure — the molecule’s energy levels — rather than its physical structure was critical to the team’s discovery. “Throwing the physical structure out the window and focusing exclusively on the electrical structure, which is something that can be performed across a range of molecular substrates, is really the crucial novel detail,” Freedman said.
In their publication, the researchers lay out the design requirements for making similar molecules, providing the framework for creating new programmable molecular qubits that can be customized for a future purpose.
“Now that we’ve established the correctness of our computational methods on these chromium qubits, we can utilize the same approaches to simplify the screening process,” said Arailym Kairalapova, one of the Columbia researchers who carried out the calculations.
“By combining chemistry and physics technologies, we can begin to comprehend the design criteria that will drive the continued advancement of this class of qubits,” Weiss explained.
Qubits that attach to biological systems could be custom-designed and used for quantum biosensing. Alternatively, researchers may design a water-soluble qubit capable of detecting signals in an aqueous environment.
“One of the fantastic things about this platform is that if the molecule doesn’t emit at a given wavelength, we can easily go back in the lab, produce a different material at a minimal cost, and evaluate which one gives us the proper feature we want,” Laorenza explained. “This is something we can complete in a matter of days. It does not necessitate a lot of intense, high-level fabrication.”
The team attributes its accomplishment to advances in the study of light-matter interactions. “A few years ago, this was just a pipe dream,” Bayliss said, “to have a set of molecular systems provide a fresh platform for quantum information science It’s incredibly thrilling to see where we are now.”
The researchers intend to investigate other ligand environments in order to broaden the range of photon emission. “This is now a launching point for many more chemists to be invited into this arena, opening up the work to a much broader variety of chemists who could contribute quite a deal to quantum information science,” Laorenza said.