One such tool that has been developed in recent years to investigate the communication between gut microbes and the brain is the use of germ-free mice. Germ-free mice are mice that are born and raised in a sterile environment, and therefore do not have any gut microbes. By comparing the behavior and physiology of germ-free mice to that of normal mice, scientists can study the effects of gut microbes on the brain. Additionally, fecal microbiota transplants (FMT) can also be used to study the effects of gut microbes on the brain, by transplanting gut microbes from a certain individual or group of individuals into germ-free or antibiotic-treated mice and observing any changes in behavior or psychiatric outcomes.
This laboratory protocol provides researchers with a road map for understanding the complex traffic system between the gut and the brain, as well as its effects in health and disease.’ Researchers have begun to recognize the significance of the gut-brain axis, a two-way communication that occurs between microbes in the gastrointestinal tract and the brain. These “conversations” can alter how these organs function, and they involve a complex network of microbe- and brain-derived chemical signals that scientists must decouple in order to gain an understanding.
“Currently, it is difficult to determine which microbial species drive specific brain alterations in a living organism,” said first author, Dr. Thomas D. Horvath, instructor of pathology and immunology at Baylor College of Medicine and Texas Children’s Hospital. “Here we present a valuable tool that enables investigations into connections between gut microbes and the brain. Our laboratory protocol allows for the identification and comprehensive evaluation of metabolites – compounds microbes produce — at the cellular and whole-animal levels.”
Gut microbes can communicate with the brain via a variety of mechanisms, including the production of metabolites such as short-chain fatty acids and peptidoglycans, neurotransmitters such as gamma-aminobutyric acid and histamine, and immune-modulating compounds.
Dr. Melinda A. Engevik
The gut microbiota is a diverse community of beneficial microorganisms found in the gastrointestinal tract. Gut microbes are increasingly being recognized for their influence on distant organs, including the brain, in addition to their roles in maintaining the intestinal environment.
“Gut microbes can communicate with the brain via a variety of mechanisms, including the production of metabolites such as short-chain fatty acids and peptidoglycans, neurotransmitters such as gamma-aminobutyric acid and histamine, and immune-modulating compounds,” said co-first author Dr. Melinda A. Engevik, assistant professor of regenerative and cellular medicine at the Medical University of South Carolina.
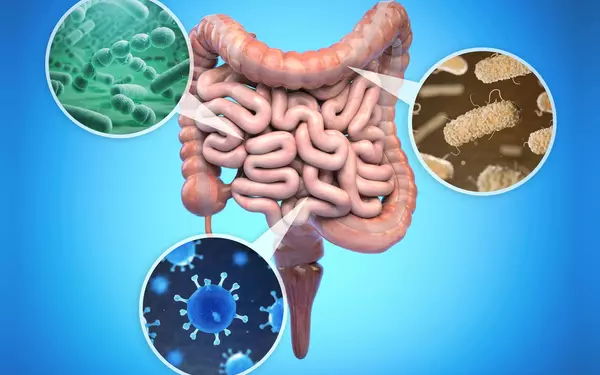
The role microbes play in the health of the central nervous system is highlighted by the links between the gut microbiome and anxiety, obesity, autism, schizophrenia, Parkinson’s disease, and Alzheimer’s disease.
“Animal models have been paramount in linking microbes to these fundamental neural processes,” said co-author Dr. Jennifer K. Spinler, assistant professor of pathology and immunology at Baylor and the Texas Children’s Hospital Microbiome Center. “The protocol in the current study enables researchers to take steps toward unraveling the specific involvement of the gut-brain axis in these conditions, as well as its role in health.”
A road map to understand the complex traffic system in the gut-brain axis
Growing the microbes in the lab first, collecting the metabolites they produced, and analyzing them using mass spectrometry and metabolomics was one strategy the researchers used to gain insight into how a single type of microbe can influence the gut and the brain. Mass spectrometry is a laboratory technique for identifying unknown compounds and quantifying known compounds by determining their molecular weight. Metabolomics is a technique for studying metabolites on a large scale.
“The effect of metabolites was then studied in mini-guts, a laboratory model of human intestinal cells that retains small intestine properties and is physiologically active,” Engevik explained. “In addition, the metabolites of the microbe can be studied in live animals.”
“We can broaden our research to include a community of microbes,” Spinler said. “In this way, we can learn about how microbial communities collaborate, synergize, and influence the host. This protocol provides researchers with a roadmap for understanding the complex traffic system between the gut and the brain, as well as its consequences.”
“This protocol was developed through large interdisciplinary collaborations involving clinicians, behavioral scientists, microbiologists, molecular biology scientists, and metabolomics experts,” Horvath said. “We hope that our approach will aid in the development of designer communities of beneficial microbes that may aid in the maintenance of a healthy body. Our protocol also provides a method for identifying potential solutions when a breakdown in communication between the gut and the brain causes disease.”