Connecting quantum computers is a difficult task since quantum information is sensitive and prone to errors. Current efforts in quantum computing networking are aimed at figuring out how to connect these devices while preserving the sensitive quantum states. Researchers have developed a new method for connecting quantum devices over great distances, paving the path for the technology to play a role in future communications networks.
Quantum transmissions, unlike today’s classical data signals, cannot be amplified across a city or an ocean. They must be repeated at regular intervals, which means they must be stopped, copied, and sent on by specialized machines known as quantum repeaters. Many scientists predict that quantum repeaters will play an important role in future communication networks, allowing for increased security and connecting remote quantum computers.
The Princeton study, published in Nature, lays the groundwork for a new technique to creating quantum repeaters. It transmits telecom-ready light produced by a single ion-implanted in a crystal. According to Jeff Thompson, the study’s lead author, the work took many years to complete. The research brought together breakthroughs in photonic design and materials science.
The gadget is composed of two components: a calcium tungstate crystal doped with only a few erbium ions and a nanoscopic piece of silicon etched into a J-shaped channel. The ion emits light up through the crystal when it is pulsed with a specific laser.
Other popular quantum repeater devices emit visible light, which degrades quickly via optical fiber and must be transformed before traveling large distances. The innovative device is based on the implantation of a single rare earth ion in a host crystal. Because this ion produces light at an optimum infrared wavelength, no signal conversion is required, resulting in simpler and more robust networks.
The gadget is composed of two components: a calcium tungstate crystal doped with only a few erbium ions and a nanoscopic piece of silicon etched into a J-shaped channel. The ion emits light up through the crystal when it is pulsed with a specific laser. But the silicon piece, a whisp of a semiconductor stuck onto the top of the crystal, catches and guides individual photons out into the fiber optic cable.
Ideally, this photon would be encoded with information from the ion, Thompson said. Or more specifically, from a quantum property of the ion called spin. In a quantum repeater, collecting and interfering the signals from distant nodes would create entanglement between their spins, allowing end-to-end transmission of quantum states despite losses along the way.
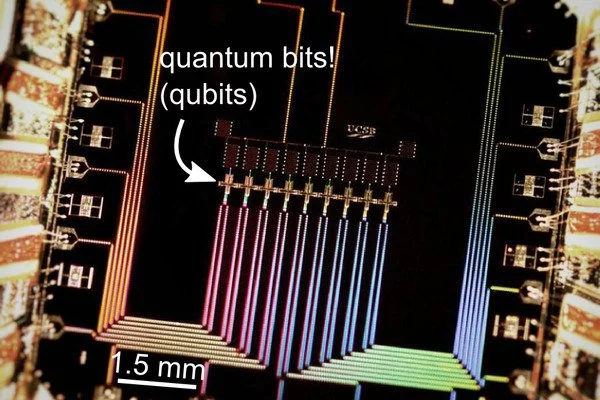
Thompson’s team first started working with erbium ions several years before, but first versions used different crystals that harbored too much noise. In particular, this noise caused the frequency of the emitted photons to jump around randomly in a process known as spectral diffusion. This prevented the delicate quantum interference that is necessary to operate quantum networks.
To solve this problem, his lab started working with Nathalie de Leon, associate professor of electrical and computer engineering, and Robert Cava, a leading solid-state materials scientist and Princeton’s Russell Wellman Moore Professor of Chemistry, to explore new materials that could host single erbium ions with much less noise.
They whittled the candidate materials list down from hundreds of thousands to a few hundred, then a couple dozen, then three. Each of the three finalists tested during a half-year period. The first content proved to be insufficiently clear. The second resulted in erbium having poor quantum characteristics. But the third, calcium tungstate, was ideal.
To show that the novel material is suited for quantum networks, the researchers constructed an interferometer in which photons are randomly routed along one of two paths: a short channel a few feet long or a large line 22 miles long (made of spooled optical fiber). Photons emitted from the ion can go on the long path or the short path, and about half the time, consecutive photons take opposite paths, and arrive at the output at the same time.
When such a collision happens, quantum interference causes photons to exit the output in pairs if and only if they are essentially indistinguishable – that is, if they have the same form and frequency. Otherwise, they depart the interferometer on their own. The team demonstrated definitively that the erbium ions in the novel material emit indistinguishable photons by measuring a high suppression (up to 80%) of individual photons at the interferometer output. That puts the signal considerably above the hi-fi threshold, according to Salim Ourari, a PhD student who co-led the research.
While this work crosses an important threshold, additional work is required to improve the storage time of quantum states in the spin of the erbium ion. The team is currently working on making more highly refined calcium tungstate, with fewer impurities that disturb the quantum spin states.