Cosmological observations of star and galaxy orbits allow for precise conclusions about the attractive gravitational forces that act between celestial bodies.
The astounding discovery: Visible matter is insufficient to explain the formation or movement of galaxies. This indicates the existence of a previously unknown type of matter. As a result, the Swiss physicist and astronomer Fritz Zwicky postulated the existence of what is now known as dark matter in 1933. Dark matter is a hypothetical form of matter that is not directly visible but interacts with us via gravity and has approximately five times the mass of matter that we are familiar with.
Recently, following a precision experiment developed at the Albert Einstein Center for Fundamental Physics (AEC) at the University of Bern, an international research team succeeded in significantly narrowing the scope for the existence of dark matter. With more than 100 members, the AEC is one of the leading international research organizations in the field of particle physics. The findings of the team, led by Bern, have now been published in Physical Review Letters.
Our experiment allows us to determine the rotational frequency of neutron spins as they move through a superposition of electric and magnetic fields. The spin of each individual neutron acts as a kind of compass needle, rotating due to a magnetic field in the same way that the second hand of a wristwatch does—but nearly 400,000 times faster.
Ivo Schulthess
The mystery surrounding dark matter
“What dark matter is actually made of is still completely unclear,” explains Ivo Schulthess, a Ph.D. student at the AEC and the lead author of the study. What is certain, however, is that it is not made from the same particles that make up the stars, planet Earth or us humans. Worldwide, increasingly sensitive experiments and methods are being used to search for possible dark matter particles—until now, however, without success.
Certain hypothetical elementary particles, known as axions, are a promising category of possible candidates for dark matter particles. An important advantage of these extremely lightweight particles is that they could simultaneously explain other important phenomena in particle physics which have not yet been understood.
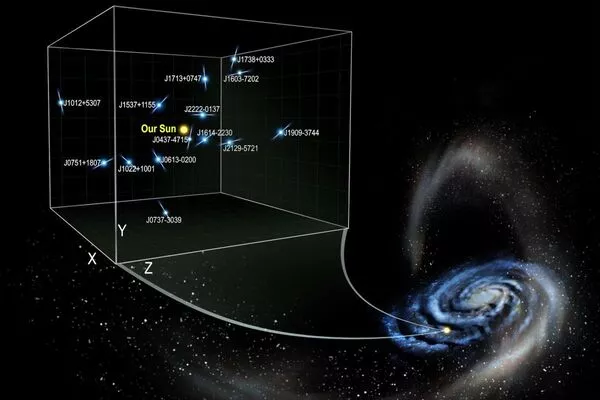
Bern experiment sheds light on the darkness
“Thanks to many years of expertise, our team has succeeded in designing and building an extremely sensitive measurement apparatus—the Beam EDM experiment,” explains Florian Piegsa, Professor for Low Energy and Precision Physics at the AEC, who was awarded one of the prestigious ERC Starting Grants from the European Research Council in 2016 for his research with neutrons. If the elusive axions actually exist, they should leave behind a characteristic signature in the measurement apparatus.
“Our experiment allows us to determine the rotational frequency of neutron spins as they move through a superposition of electric and magnetic fields,” Schulthess explains. The spin of each individual neutron acts as a kind of compass needle, rotating due to a magnetic field in the same way that the second hand of a wristwatch does—but nearly 400,000 times faster.
“We precisely measured this rotational frequency and examined it for the smallest periodic fluctuations that would be caused by interactions with the axions,” Piegsa explains. “The rotational frequency of the neutrons remained unchanged, indicating that there is no evidence of axions in our measurement,” Piegsa says.
Parameter space successfully narrowed down
The measurements, which were carried out with researchers from France at the European Research Neutron Source at the Institute Laue-Langevin, allowed for the experimental exclusion of a previously completely unexplored parameter space of axions. It also proved possible to search for hypothetical axions which would be more than 1,000 times heavier than was previously possible with other experiments.
“Although the existence of these particles remains mysterious, we have successfully excluded an important parameter space of dark matter,” concludes Schulthess. Future experiments can now build on this work. “Finally answering the question of dark matter would give us a significant insight into the fundamentals of nature and take us a big step closer to a complete understanding of the universe,” explains Piegsa.