The mitochondrion has a well-deserved reputation as the “powerhouse of the cell.” These tiny but powerful organelles perform a variety of life-sustaining functions, ranging from powering our own cells and organs to fueling chemical and biological processes. However, when they aren’t working properly, a variety of rare diseases can develop.
Mitochondrial diseases are a group of debilitating genetic disorders that affect one in every 5,000 people worldwide, the majority of whom are children. With these diseases come a slew of health issues, including, but not limited to, heart disease, developmental and cognitive disabilities, respiratory problems, stunted growth, and even premature death. As of right now, there is no cure.
However, recent research published in the journals Mitochondrion and BMC Molecular and Cell Biology by Aloka Abey Bandara, a research associate professor in the Department of Biomedical Sciences and Pathobiology at the Virginia-Maryland College of Veterinary Medicine, and his team provides mitochondrial disease patients and their parents with a ray of hope.
Bandara has successfully created live cell models that mimic mitochondrial disease cells in collaboration with a team of Virginia Tech researchers from Blacksburg and Roanoke. These cells will lay the groundwork for future drug studies and mitochondrial disease research.
“Our cell models will enable us to see exactly what happens to the cells and their processes when a child develops the mitochondrial disease. In addition to these considerations, we will be able to conduct additional research into the toxicity and efficacy of novel drug candidates “Bandara, who is also a member of the Fralin Life Sciences Institute’s affiliated faculty, explained.
The food we eat and the air we breathe provide our bodies with life-sustaining energy. Oxygen and nutrients, such as glucose, travel through the organs, tissues, and cells of the body until they reach their final destination: the mitochondria. When nutrients reach the inner membrane of the mitochondria, a distinct series of protein complexes known as the electron transport chain is activated.
The mitochondrion has garnered quite the reputation for its role as the ‘powerhouse of the cell.’ These tiny, but mighty organelles play various life-sustaining roles, from powering our own cells and organs to fueling chemical and biological processes.
The electron transport chain is able to remove electrons from nutrients and push them through the mitochondrial membrane, forming a gradient of protons, through a series of reactions. When this happens, the body produces adenosine triphosphate, or ATP, a molecule that transports energy within cells.
“Occasionally, you can see disruptions or mutations within the electron transport chain proteins,” Bandara explained. “As a result, the protein complexes are unable to transport electrons, causing energy production to be disrupted. Almost all organs of the organism will be affected, including the heart, eyes, and muscles, and will be unable to function properly.”
The electron transport chain is made up of five protein complexes, or protein groups. Complex I and Complex II are two protein complexes that are in charge of removing electrons from nutrients. If they fail to perform their duties, the entire electron transport chain fails, and the body is unable to produce ATP.
Patients with mitochondrial disease may have defects in either Complex I or Complex II. Patients with Complex I disruptions are more likely to have neurological issues, such as seizures and abnormal brain functions. Those who have Complex II disruptions are more likely to develop a variety of diseases, including cancer.
Although researchers have pinpointed the exact location of the defects, developing treatments for these mitochondrial diseases has proven difficult. Therapies, vitamins, and dietary changes have been shown to help alleviate symptoms and slow disease progression; however, the mitochondrial disease does not have a cure. As a result, new drugs must be developed, tested, and refined.
Bandara hopes that his cell lines will help not only future research but also patients and their families who are dealing with mitochondrial disease and all of its consequences firsthand.
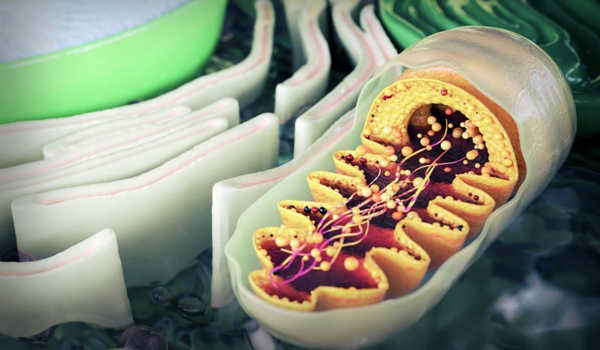
“Parents are frequently helpless because they cannot simply go to the pharmacy and get a drug,” Bandara explained. “They should be able to see that Virginia Tech is making significant strides toward finding a cure for these diseases. Perhaps they can sense that they are no longer alone, that universities, the government, and science are fighting alongside them.”
Researchers must first create cellular models that act as artificial “sick” cells in order to test drug candidates. Because the mitochondrial disease can be studied without removing cells from patients, cellular models are an excellent tool for drug discovery.
Bandara used CRISPR/Cas9 technology to “knock out” portions of the genome that code for Complex I and Complex II in order to create cells that mimic mitochondrial disease. First, the researchers determined which parts of the genome needed to be removed. Then they created a piece of RNA that designated that point as its “home base.” The RNA then “guided” a Cas9 enzyme to its home base on the gene. Cas9 can then bind to that point and “cut” it.
Following the completion of this process, Bandara performed genomic sequencing to confirm that the portion of the genome had been successfully deleted. Bandara and his colleagues worked for months to develop two mutant cell lines, one with Complex I removed and the other without Complex II. Bandara is one of only a few researchers on the Virginia Tech Blacksburg campus using CRISPR/Cas9 technology to treat mitochondrial disease.
After creating the mutant cell lines, Bandara put them through a disease model, testing the functions of the “sick” cell line against the “parent” cell line, which is made up of healthy cells. Bandara confirmed that the sick cells consumed far less oxygen, grew slowly, and did not produce enough ATP for the cells to function properly – the three hallmarks of mitochondrial disease cells.
They were able to test a newly developed drug called Idebenone after confirming that the knockout cell lines accurately simulated the cellular dysfunctions of mitochondrial disease. Bandara demonstrated that cell growth and oxygen consumption can be restored to some extent with this treatment. Experts from the Department of Human Nutrition, Foods, and Exercise and the Virginia Tech Carilion School of Medicine collaborated to create these cell lines.
David Brown, a former associate professor in the Department of Human Nutrition, Foods, and Exercise in the Virginia Tech College of Agriculture and Life Sciences who is now the senior director of scientific and technical innovation at Stealth BioTherapeutics, a Boston-based biotechnology company, guided and supported the development of mutant cell lines.
The team has received two provisional patents for their cells as a result of their efforts. One of the cell lines has already been patented and licensed to a pharmaceutical company, which will use it to create new therapies for people with mitochondrial diseases.
Through Ximbio, the world’s largest nonprofit specializing in life science research tools of all types, these cells have been made available for global use by interested researchers and pharmaceutical companies.
“Cell models of mitochondrial Complex I and II defects have a high societal and economic impact as models for testing drug candidates in a cost- and time-effective manner for the treatment of mitochondrial dysfunction,” said Justin Perry, a Virginia Tech graduate who now works as a business development manager at Ximbio.