Gases can be imaged in rainbow colors through a technique called spectroscopy. Spectroscopy is the study of the interaction of electromagnetic radiation with matter. When electromagnetic radiation, such as light, passes through a gas, the gas atoms or molecules absorb certain wavelengths of the radiation and emit others. The absorbed and emitted wavelengths of radiation correspond to specific colors, which can be captured and recorded by a spectrograph. This creates a “spectrum” of colors that can be used to identify the specific types of atoms or molecules in the gas.
Additionally, there is a technique known as “laser-induced fluorescence” where a laser is used to excite atoms or molecules, causing them to emit light at specific wavelengths. The emitted light can be separated into its individual colors by a spectrograph, creating a “rainbow” image of the gas. This technique is commonly used in laboratory experiments, but also in atmospheric research or chemical industries to identify, trace and quantify the gases.
Scientists created a simple device capable of imaging a gas injected into it in multiple colors based on its gaseous properties, allowing for chromatic discrimination of different gases. This simple device converts the pressure generated by an injected gas into structural colors, allowing it to be imaged. This technology has the potential to be used in a variety of fields, including environmental monitoring, safety assurance, and healthcare.
Imaging of gases is important in many gas-related basic and applied research projects as almost all ambient gases are colorless and invisible. Only a few methods for imaging ambient gas flow have been developed.
NIMS, Harvard University, and the University of Connecticut created a simple device that can image a gas injected into it in multiple colors based on its gaseous properties, allowing for chromatic discrimination of different gases. This simple device converts the pressure generated by an injected gas into structural colors, allowing it to be imaged. This technology has the potential to be used in a variety of fields, including environmental monitoring, safety assurance, and healthcare.
Imaging of gases is important in many gas-related basic and applied research projects as almost all ambient gases are colorless and invisible. Only a few methods for imaging ambient gas flow have been developed (e.g., the use of infrared cameras capable of detecting temperature changes and air flow measurements by means of releasing tracer particles into the air).
These methods require elaborate equipment and are unsuitable for imaging different types of gases in a consistent manner. In addition, the images they produce are unfit for the analysis of gaseous characteristics. A simple method capable of imaging and analyzing all types of gases may have a wide variety of applications, such as image-based measurements.
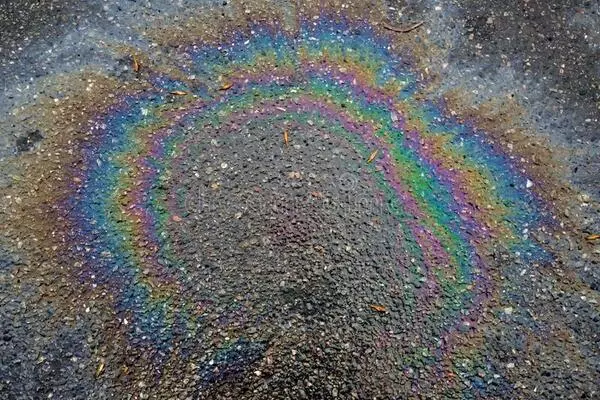
This research team recently fabricated a device capable of imaging and differentiating various gases using a wide range of colors (i.e., structural colors) through a simple procedure: polydimethylsiloxane (PDMS) – a soft material – was first shaped into a slab. Part of the PDMS surface was then treated with argon plasma.
The plasma-treated PDMS slab was placed with its plasma-treated surface down on the surface of a glass substrate, and they made complete contact. When compressed by an injected gas passing through the tight boundary between the PDMS and glass layers, the plasma-treated PDMS surface forms a periodic ripple-like micropattern. This compression and resultant micropattern formation lead to the production of structural colors. This mechanism can be used to image and differentiate any type of gas.
When the incoming gas flow is interrupted, structural colors vanish completely. The degree of PDMS deformation is determined by the injected gases’ flow rates, viscosities, and densities. Because all gases have different viscosities and densities, this device can be used to differentiate and analyze gas samples at a constant flow rate based on these properties.
In the future, the team will work to improve the device’s sensitivity in order to make it compatible with a variety of applications (e.g., identification of ambient gases and biological samples). The team will also consider developing a new gas identification technique by combining it with image recognition and machine learning techniques, as well as fabricating a small, CCD-integrated device with a simple structure.