Engineers and materials scientists investigating superconducting quantum information bits (qubits), a major quantum computing material platform based on the frictionless flow of paired electrons, have discovered hints to the microscopic origins of qubit information loss. This loss is one of the most significant impediments to the development of quantum computers capable of stringing together millions of qubits to perform complex calculations.
Such enormous scope, shortcoming lenient frameworks could mimic muddled atoms for drug improvement, speed up the revelation of new materials for clean energy, and perform different undertakings that would be inconceivable or require an illogical measure of time (a long period of time) for the present most remarkable supercomputers.
Superconducting qubits are a promising quantum computing platform because we can engineer their properties and make them using the same tools used to make regular computers.
Anjali Premkumar
The nature of the atomic-scale imperfections that lead to qubit information loss is currently poorly understood. The team used state-of-the-art characterization capabilities at the Center for Functional Nanomaterials (CFN) and National Synchrotron Light Source II (NSLS-II), both DOE Office of Science User Facilities at Brookhaven National Laboratory, to help bridge the gap between material properties and qubit performance. Their findings identified structural and surface chemical flaws in superconducting niobium qubits that might be the source of loss.
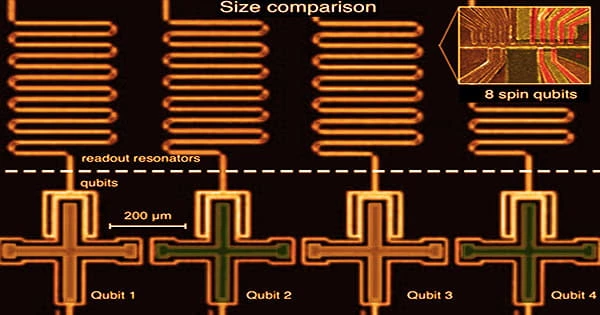
“Superconducting qubits are a promising quantum computing platform because we can engineer their properties and make them using the same tools used to make regular computers,” said Anjali Premkumar, a fourth-year graduate student in the Houck Lab at Princeton University and first author on the Communications Materials paper describing the research. “However, they have shorter coherence times than other platforms.”
To put it another way, they can’t keep knowledge for very long before losing it. Though single qubit coherence times have recently increased from microseconds to milliseconds, when numerous qubits are linked together, these periods substantially drop.
“Qubit coherence is limited by the quality of the superconductors and the oxides that will inevitably grow on them as the metal comes into contact with oxygen in the air,” continued Premkumar. “But, as qubit engineers, we haven’t characterized our materials in great depth. Here, for the first time, we collaborated with materials experts who can carefully look at the structure and chemistry of our materials with sophisticated tools.”
The Co-design Center for Quantum Advantage (C2QA), one of five National Quantum Information Science Centers formed in 2020 to support the National Quantum Initiative, was a “prequel” to this partnership. C2QA, led by Brookhaven Lab, brings together hardware and software engineers, physicists, materials scientists, theorists, and other specialists from national laboratories, universities, and businesses to overcome quantum hardware and software performance concerns.
The C2QA team aims to understand and eventually control material properties to extend coherence times, design devices to generate more robust qubits, optimize algorithms to target specific scientific applications, and develop error-correction solutions through co-design efforts in materials, devices, and software.
The researchers used three distinct sputtering processes to create thin niobium metal sheets in their investigation. Sputtering is a process in which energetic particles are fired at a target containing the desired material, causing atoms to eject from the target and drop on a neighboring substrate. The Houck Lab used traditional (direct current) sputtering, whereas Angstrom Engineering used a novel type of sputtering (high-power impulse magnetron sputtering, or HiPIMS), which involves striking the target with brief bursts of high-voltage energy.
HiPIMS was tested in two ways by Angstrom: normal and with optimized power and target-substrate geometry. Premkumar used the three sputtered films to create “transmon” qubit devices, which he stored in a dilution cooler back at Princeton.
Temperatures inside this refrigerator may drop to near absolute zero (minus 459.67 degrees Fahrenheit), causing qubits to become superconducting. Superconducting pairs of electrons tunnel via an insulating barrier of aluminum oxide (Josephson junction) sandwiched between superconducting aluminum layers, which are connected to niobium on sapphire capacitor pads in these devices.
As electron pairs pass from one side of the barrier to the other, the qubit state changes. Because they are highly insensitive to fluctuations in electric and magnetic fields in the surrounding environment, transmon qubits, co-invented by Houck Lab principal investigator and C2QA Director Andrew Houck, are a leading type of superconducting qubit. Such fluctuations can cause qubit information loss.
Premkumar assessed the energy relaxation time for each of the three device types, which is a measure of the qubit state’s resilience.
“The energy relaxation time corresponds to how long the qubit stays in the first excited state and encodes information before it decays to the ground state and loses its information,” explained Ignace Jarrige, formerly a physicist at NSLS-II and now a quantum research scientist at Amazon, who led the Brookhaven team for this study.
The relaxation periods of each device were varied. The researchers used microscopy and spectroscopy at the CFN and NSLS-II to further comprehend these distinctions.
Scientists at the NSLS-II beamline used x-ray photoemission spectroscopy with soft x-rays at the In situ and Operando Soft X-ray Spectroscopy (IOS) beamline and hard x-rays at the Spectroscopy Soft and Tender (SST-2) beamline to detect the oxidation states of niobium. They discovered several suboxides between the metal and the surface oxide layer that had a lower oxygen content than niobium through these spectroscopic experiments.
“We needed the high energy resolution at NSLS-II to distinguish the five different oxidation states of niobium and both hard and soft x-rays, which have different energy levels, to profile these states as a function of depth,” explained Jarrige. “Photoelectrons generated by soft x-rays only escape from the first few nanometers of the surface, while those generated by hard x-rays can escape from deeper in the films.”
The scientists used resonant inelastic x-ray scattering at the NSLS-II Soft Inelastic X-ray Scattering (SIX) beamline to find regions with missing oxygen atoms (RIXS). Defects in the form of oxygen vacancies can absorb energy from qubits.
The researchers used transmission electron microscopy and atomic force microscopy at the CFN to examine film shape and electron energy-loss spectroscopy to assess the local chemical composition at the film surface.
“The microscope images showed grains pieces of individual crystals with atoms arranged in the same orientation sized larger or smaller depending on the sputtering technique,” explained coauthor Sooyeon Hwang, a staff scientist in the CFN Electron Microscopy Group.
“The smaller the grains, the more grain boundaries, or interfaces where different crystal orientations meet. According to the electron energy-loss spectra, one film had not just oxides on the surface but also in the film itself, with oxygen diffused into the grain boundaries.”
The researchers discovered relationships between qubit relaxation periods and the number and breadth of grain boundaries, as well as the concentration of suboxides near the surface, in their experiments at the CFN and NSLS-II.
“Grain boundaries are defects that can dissipate energy, so having too many of them can affect electron transport and thus the ability of qubits to perform computations,” said Premkumar. “Oxide quality is another potentially important parameter. Suboxides are bad because electrons are not happily paired together.”
The team will continue to work together in the future to better understand qubit coherence using C2QA. One line of inquiry is to see if relaxation periods may be shortened by improving fabrication procedures to produce films with bigger grain sizes (i.e., fewer grain boundaries) and a single oxidation state. They’ll also look into other superconductors, such as tantalum, which has more chemically homogenous surface oxides.
“From this study, we now have a blueprint for how scientists who make qubits and scientists who characterize them can collaborate to understand the microscopic mechanisms limiting qubit performance,” said Premkumar. “We hope other groups will leverage our collaborative approach to drive the field of superconducting qubits forward.”
The DOE Office of Science, the National Science Foundation Graduate Research Fellowship, the Humboldt Foundation, the National Defense Science and Engineering Graduate Fellowship, the Materials Research Science and Engineering Center, and the Army Research Office all contributed to this research.
The Electron Microscopy, Proximal Probes, and Theory and Computation Facilities of the CFN, a DOE Nanoscale Science Research Center, were employed in this study. The National Institute of Standards and Technology runs the SST-2 beamline at NSLS-II.