The Hubble Space Telescope is primarily an optical telescope and it observes the universe by capturing light from distant objects. It is not capable of directly measuring the mass of a star or any other object. However, astronomers can indirectly estimate the mass of a white dwarf by observing its gravitational influence on other objects in its vicinity. For example, a white dwarf in a binary star system can cause its companion star to orbit around it, and by measuring the orbital period and the separation between the two stars, astronomers can calculate the mass of the white dwarf.
The mass of a single, isolated white dwarf – the surviving core of a burned-out, Sun-like star – has been directly measured by astronomers. The white dwarf is 56 percent the mass of our Sun, according to researchers. This agrees with previous theoretical predictions of the white dwarf’s mass and supports current theories of how white dwarfs evolve as the end product of the evolution of a typical star. The one-of-a-kind observation adds to theories about the structure and composition of white dwarfs.
For the first time, astronomers using NASA’s Hubble Space Telescope directly measured the mass of a single, isolated white dwarf — the surviving core of a burned-out, Sun-like star. The white dwarf is 56 percent the mass of our Sun, according to researchers. This agrees with previous theoretical predictions of the white dwarf’s mass and supports current theories of how white dwarfs evolve as the end product of the evolution of a typical star. The one-of-a-kind observation adds to theories about the structure and composition of white dwarfs.
Previously, white dwarf mass measurements were obtained by observing white dwarfs in binary star systems. Simple Newtonian physics can be used to calculate the masses of two co-orbiting stars by observing their motion. However, if the white dwarf’s companion star has a long period of hundreds or thousands of years, these measurements may be uncertain. Telescopes can only measure orbital motion over a small portion of the dwarf’s orbital motion.
The precision of LAWD 37’s mass measurement allows us to test the mass-radius relationship for white dwarfs. This means testing the theory of degenerate matter under the extreme conditions inside this dead star.
Peter McGill
For this companion-less white dwarf, researchers had to use a natural phenomenon known as gravitational microlensing. The light from a background star was slightly deflected by the foreground dwarf star’s gravitational warping of space. As the white dwarf passed in front of the background star, microlensing caused the star to appear temporarily offset from its actual position on the sky.
The findings were published in the Royal Astronomical Society’s Monthly Notices. Peter McGill, formerly of the University of Cambridge, is the lead author (now based at the University of California, Santa Cruz). McGill used Hubble to precisely measure how light from a distant star bent around the white dwarf, known as LAWD 37, causing the background star’s apparent position in the sky to change temporarily.
The principal Hubble investigator on this latest observation, Kailash Sahu of the Space Telescope Science Institute in Baltimore, Maryland, first used microlensing in 2017 to measure the mass of another white dwarf, Stein 2051 B. However, that dwarf is part of a widely separated binary system. “Our latest observation provides a new benchmark because LAWD 37 is all by itself,” Sahu said.
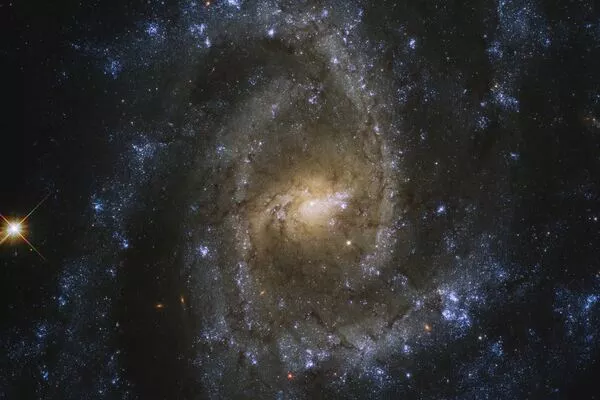
LAWD 37, the collapsed remains of a star that died 1 billion years ago, has been extensively studied due to its proximity to Earth (15 light-years away in the constellation Musca). “Because this white dwarf is relatively close to us, we have a lot of data on it — we have information about its light spectrum, but the missing piece of the puzzle has been a measurement of its mass,” McGill explained.
The ESA’s Gaia space observatory, which makes extraordinarily precise measurements of nearly 2 billion star positions, helped the team zero in on the white dwarf. A star’s motion can be tracked using multiple Gaia observations. Based on this data, astronomers were able to predict that LAWD 37 would briefly pass in front of a background star in November 2019.
Once this was known, Hubble was used to precisely measure over several years how the background star’s apparent position in the sky was temporarily deflected during the white dwarf’s passage.
“These events are rare, and the effects are tiny,” said McGill. “For instance, the size of our measured offset is like measuring the length of a car on the Moon as seen from Earth.”
Since the light from the background star was so faint, the main challenge for astronomers was extracting its image from the glare of the white dwarf, which is 400 times brighter than the background star. Only Hubble can make these kinds of high-contrast observations in visible light.
“The precision of LAWD 37’s mass measurement allows us to test the mass-radius relationship for white dwarfs,” said McGill. “This means testing the theory of degenerate matter (a gas so super-compressed under gravity it behaves more like solid matter) under the extreme conditions inside this dead star,” he added.
According to the researchers, their findings pave the way for future event prediction using Gaia data. These alignments can now be detected by NASA’s James Webb Space Telescope in addition to Hubble. Because Webb works at infrared wavelengths, the blue glow of a foreground white dwarf appears dimmer, while the background star appears brighter.
Sahu is using NASA’s James Webb Space Telescope to observe another white dwarf, LAWD 66, based on Gaia’s predictions. The first observation occurred in 2022. More observations will be made as the deflection reaches a peak in 2024 and then declines.
“Gaia has really changed the game – it’s exciting to be able to use Gaia data to predict when events will happen, and then observe them happening,” said McGill. “We want to continue measuring the gravitational microlensing effect and obtain mass measurements for many more types of stars.”
Einstein predicted in his 1915 theory of general relativity that when a massive compact object passes in front of a background star, the light from the star will bend around the foreground object due to the warping of space caused by the star’s gravitational field.
Exactly a century before Hubble’s latest discovery, two British-organized expeditions to the southern hemisphere discovered this lensing effect during a solar eclipse on May 19th, 1919. It was heralded as the first experimental proof of general relativity, which states that gravity warps space. However, because of the precision required, Einstein was skeptical that the effect could ever be detected for stars outside our solar system. “Our measurement is 625 times smaller than the effect observed during the 1919 solar eclipse,” McGill said.