To estimate biomolecular motor operating models from single-molecule imaging data of motion using the Bayesian inference framework, an unique mathematical modeling technique has been created.
By mathematically modeling experimental imaging data, the mechanism of a linear molecular motor called a “chitinase,” which goes one direction on a chitin chain while degrading the chain passed by, was clarified.
Cells’ biomolecular motors produce unidirectional motion by utilizing chemical energy obtained from processes like the hydrolysis of ATP. It has garnered a lot of interest to clarify the workings of these molecular motors, which are protein-based nanomachines created by nature. A promising method for figuring out how molecular motors work is single-molecule imaging, which can record the motion of molecules in real time.
However, it is still unknown how the unidirectional motion of the whole motors is caused by the consumption of chemical energy, i.e., a change in the chemical states of such motor proteins. Researchers from the Institute for Molecular Science and Shizuoka University have discovered that variations in the chemical state of a molecular motor cause changes in the morphologies of the free energy profiles along the motion of the motor.
To describe how the molecular motors move, the researchers initially tried to create a computational model. It is possible to think of a motor’s motion as diffusive motion on free energy profiles that change depending on the chemical states of the molecules that make up the motor.
We will apply our method developed in this study to various molecular motors and hope to clarify the similarities and differences in the mechanisms of the molecular motors. We believe that new findings will be obtained by our method in the future and give us a clue to the general operation principles of molecular motors. Studies using our method will pave the way for designing new artificial molecular motors.
Kei-ichi Okazaki
More specifically, as shown in Fig. 1A, the motor first moves on the free energy surface of chemical state 1 (red) of the motor molecules, and then moves on the free energy surface of chemical state 2 (blue).
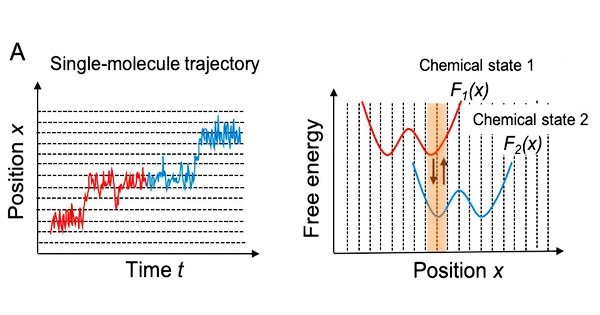
However, single-molecule imaging seldom captures this chemical state change. The researchers used a hidden Markov model, in which the chemical states are thought of as “hidden,” to treat the transition between them.
The “likelihood,” which assesses the probability to demonstrate how well the model explains the course of the real single-molecule motion, can be calculated using this hidden Markov model. It is also feasible to use prior probabilities that are informed by the free energy profiles.
By using Monte Carlo sampling with posterior probabilities expressed as a product of the likelihood and the prior probabilities, the researchers have developed a method for estimating chemical-state-dependent free energy profiles, diffusion coefficients on each profile, and rate constants of transitions between these states within the Bayesian inference framework.
Then, using single-molecule imaging, the method created in the current study was used to examine the motion of the linear molecular motor chitinase. The characteristic free energy profiles that control the motion were discovered by analyzing the trajectory data of chitinase’s unidirectional motion while degrading a chitin chain. The analysis’s findings demonstrated that a chitinase crosses a relatively low free energy barrier using Brownian motion to travel along a rail of chitin chain.
Then, the chitin chain is hydrolyzed and the reaction products are dissociated to swap chemical states, resulting in the unidirectional motion. The “burnt-bridge” Brownian ratchet mechanism that the researchers have previously reported has a physical foundation thanks to the current discovery.
“We will apply our method developed in this study to various molecular motors and hope to clarify the similarities and differences in the mechanisms of the molecular motors. We believe that new findings will be obtained by our method in the future and give us a clue to the general operation principles of molecular motors. Studies using our method will pave the way for designing new artificial molecular motors,” said Okazaki.