A worldwide group of researchers, driven by astrophysicists from the University of Bath in the UK, has estimated the magnetic field in a far-away Gamma-Ray Burst (GRB), affirming interestingly a decades-in length hypothetical expectation that the magnetic field in these impact waves becomes mixed after the launched out material collides with, and shocks, the encompassing medium.
In the breakdown of radioactive atomic nuclei and the decay of some subatomic particles, gamma rays are created. The energies of gamma-ray photons have no theoretical upper limit, and the wavelengths of these extremely high-energy photons have no theoretical lower limit; observed energies currently extend up to a few trillion electron volts. These extremely high-energy photons are produced in astronomical sources through currently unknown mechanisms.
This new study builds on our research that has shown the most powerful GRBs can be powered by large-scale ordered magnetic fields, but only the fastest telescopes will catch a glimpse of their characteristic polarization signal before they are lost to the blast.
Ms Jordana-Mitjans
According to NASA, the longer-lived variants of gamma-ray bursts have been linked to extraordinarily strong supernovas known as hyper novas, which occur when stars between five and ten times the mass of our sun die and implode into black holes. The effects of freed atomic electrons in gases, crystals, and semiconductors are used in standard gamma-ray detection methods.
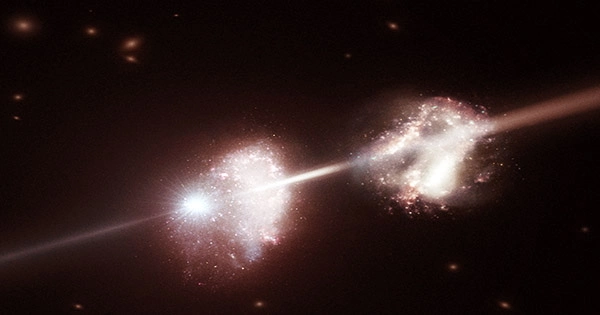
When enormous stars (at least 40 times the size of our Sun) die in a cataclysmic explosion that generates a blast wave, black holes arise. Gamma-Ray Bursts (GRBs) are very intense events that eject material at near-light speeds and produce brilliant, short-lived gamma-ray flashes that may be detected by satellites orbiting the Earth, thus its name.
Magnetic fields may be threaded through the expelled material, and these magnetic fields twist into corkscrew shapes as the spinning black hole grows, focusing and speeding up the ejected material.
The magnetic fields are invisible, but their trace is recorded in the light emitted by charged particles (electrons) whizzing about the magnetic field lines. This light, which has traveled millions of years across the Universe, is captured by Earth-based telescopes.
Gamma-ray bursts also appear to focus their energy in a narrow beam rather than discharging it evenly in all directions, which means that many of them are missed by our satellites. Although satellites detect only one gamma-ray burst every day, astronomers believe that 500 occur in the same time frame.
Head of Astrophysics at Bath and gamma-ray expert Professor Carole Mundell, said: “We measured a special property of the light polarization to directly probe the physical properties of the magnetic field powering the explosion. This is a great result and solves a long-standing puzzle of these extreme cosmic blasts a puzzle I’ve been studying for a long time.”
Capturing the Light Early
The goal is to catch the light as quickly as possible after a burst and decode the physics of the explosion, with the prediction that any primordial magnetic fields will be annihilated when the expanding shock front collides with star debris.
When the large-scale primordial field is still intact and driving the outflow, this model predicts light with high levels of polarization (>10 percent). As the field is disrupted in the impact, the light should be mainly unpolarized afterward.
Minutes after the burst, Mundell’s team discovered strongly polarized light, confirming the presence of primordial fields with large-scale structure. However, the prognosis for expanding forward shocks has been more divisive.
Teams that monitored GRBs in the hours to days following a burst discovered little polarization and concluded that the fields had been destroyed long ago, but they couldn’t explain when or how. A group of Japanese astronomers, on the other hand, revealed the discovery of 10% polarized light in a GRB, which they interpreted as a polarized forward shock with long-lasting organized magnetic fields.
Lead author of the new study, Bath PhD student Nuria Jordana-Mitjans, said: “These rare observations were difficult to compare, as they probed very different timescales and physics. There was no way to reconcile them in the standard model.”
The mystery remained unsolved for over a decade until the Bath team’s analysis of GRB 141220A.
Professor Mundell’s team reports the discovery of very low polarization in forward-shock light discovered barely 90 seconds after the detonation of GRB 141220A in a new study published today in the Monthly Notices of the Royal Astronomical Society. The team’s intelligence software on the completely autonomous robotic Liverpool Telescope and the innovative RINGO3 polarimeter, which documented the GRB’s color, brightness, polarization, and rate of fading, enabled the super-fast observations. Putting together this data, the team was able to prove that:
- The light originated in the forward shock.
- The magnetic field length scales were much smaller than the Japanese team inferred.
- The outburst was most likely fueled by the breakdown of organized magnetic fields in the early stages of a new black hole’s development.
- A contribution of polarized light from the primordial magnetic field before it was annihilated in the shock might explain the puzzling observation of polarization by Japanese researchers.
Ms Jordana-Mitjans said: “This new study builds on our research that has shown the most powerful GRBs can be powered by large-scale ordered magnetic fields, but only the fastest telescopes will catch a glimpse of their characteristic polarization signal before they are lost to the blast.”
Professor Mundell added: “We now need to push the frontiers of technology to probe the earliest moments of these blasts, capture statistically significant numbers of bursts for polarization studies and put our research into the wider context of real-time multi-messenger follow-up of the extreme Universe.”